Thank you for visiting nature.com. You are using a browser version with limited support for CSS. To obtain the best experience, we recommend you use a more up to date browser (or turn off compatibility mode in Internet Explorer). In the meantime, to ensure continued support, we are displaying the site without styles and JavaScript.
- View all journals
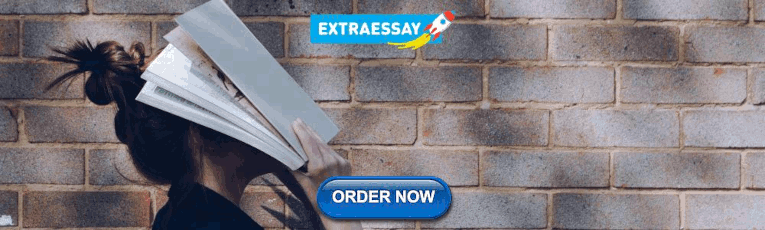
Malaria articles from across Nature Portfolio
Malaria is a mosquito-borne disease that is caused by Plasmodium parasites. Patients with malaria experience flu-like symptoms and, in severe cases, the disease can progress to neurological disturbances, coma and death. Malaria is endemic in tropical and subtropical regions and causes up to one million deaths each year.
Latest Research and Reviews
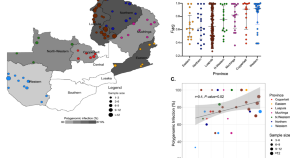
Genomics reveals heterogeneous Plasmodium falciparum transmission and selection signals in Zambia
Fola et al . perform a nationwide genomic surveillance study of P. falciparum parasites across Zambia from samples collected in 2018 and report key genetic metrics informing transmission intensity, genetic relatedness, and selection. These genomic surveillance data highlight the need to strengthen malaria control and surveillance of drug resistance.
- Abebe A. Fola
- Giovanna Carpi
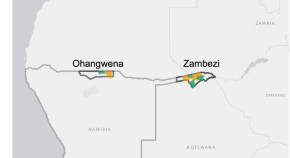
Estimating the size of populations at risk for malaria: a case study in cattle herders and agricultural workers in Northern Namibia
- Francois Rerolle
- Jerry O. Jacobson
- Jennifer L. Smith
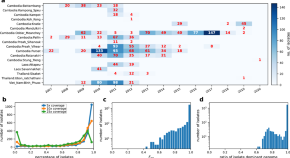
Strong positive selection biases identity-by-descent-based inferences of recent demography and population structure in Plasmodium falciparum
Identity-by-descent (IBD) is used to infer malaria parasite population demography, but positive selection imposed by drug pressure can bias IBD estimates. This study shows that strong selection distorts IBD distributions impacting downstream inferences and presents an approach to correct these biases.
- Victor Borda
- Shannon Takala-Harrison
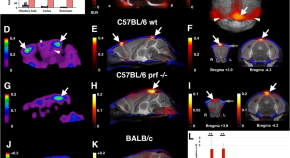
Beyond the microcirculation: sequestration of infected red blood cells and reduced flow in large draining veins in experimental cerebral malaria
Cerebral malaria can lead to fatal brain swelling. Oelschlegel et al. find infected red blood cells and reduced flow in large draining cerebral veins in mouse model of cerebral malaria, suggesting that impaired venous efflux could cause the swelling.
- A. M. Oelschlegel
- R. Bhattacharjee
- G. Nishanth
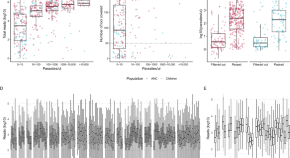
Genomic malaria surveillance of antenatal care users detects reduced transmission following elimination interventions in Mozambique
Routine sampling of pregnant women at first antenatal care (ANC) visits could be used for malaria surveillance. Here, the authors compare the genetic structure of Plasmodium falciparum parasite populations between samples from first ANC users and children from the community in Mozambique, and show that it can inform about changes in transmission beyond epidemiological data.
- Nanna Brokhattingen
- Glória Matambisso
- Alfredo Mayor
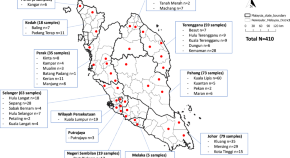
The prevalence of simian malaria in wild long-tailed macaques throughout Peninsular Malaysia
- Shahhaziq Shahari
- Mohd Lutfi Bin Abdullah
- Yee Ling Lau
News and Comment
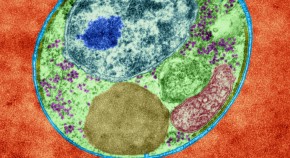
Ancient malaria genome from Roman skeleton hints at disease’s history
Genetic information from ancient remains is helping to reveal how malaria has moved and evolved alongside people.
- Tosin Thompson
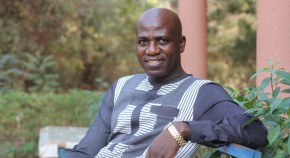
Malaria fighter: this researcher paved the way for a game-changing vaccine
Halidou Tinto runs a clinic in rural Burkina Faso that has been instrumental to the approval of the world’s first malaria vaccines.
- Brendan Maher
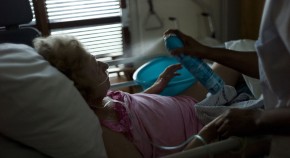
Climate change is also a health crisis — these 3 graphics explain why
Health is on the agenda at the COP28 climate meeting. Rising temperatures increase the spread of infectious diseases, claim lives and drive food insecurity.
- Carissa Wong
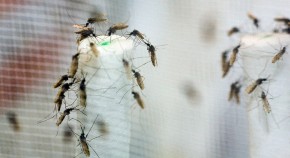
Half a million children die of malaria every year. Finally we can change that
With two vaccines available, this killer disease could now be eliminated — but will the world pull together to make it happen?
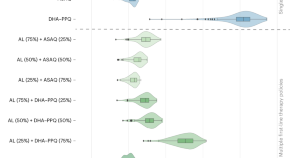
Modeling to guide drug policy response to artemisinin-resistant malaria in Rwanda
A spatially coupled, individual-based simulation of malaria in Rwanda was used to evaluate changes in drug policy in response to artemisinin-resistant Plasmodium falciparum . Our findings suggest that the deployment of multiple first-line therapies has the potential to reduce treatment failures and slow the fixation of resistant alleles in populations.
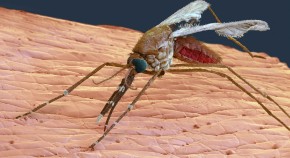
Second malaria vaccine to win global approval is cheaper and easier to make
The World Health Organization has recommended a shot called R21 to prevent the disease in children.
- Miryam Naddaf
Quick links
- Explore articles by subject
- Guide to authors
- Editorial policies

- Open access
- Published: 12 July 2016
The development of malaria diagnostic techniques: a review of the approaches with focus on dielectrophoretic and magnetophoretic methods
- Surasak Kasetsirikul 1 ,
- Jirayut Buranapong 1 ,
- Werayut Srituravanich 1 ,
- Morakot Kaewthamasorn 2 &
- Alongkorn Pimpin ORCID: orcid.org/0000-0003-4397-3948 1
Malaria Journal volume 15 , Article number: 358 ( 2016 ) Cite this article
11k Accesses
57 Citations
6 Altmetric
Metrics details
The large number of deaths caused by malaria each year has increased interest in the development of effective malaria diagnoses. At the early-stage of infection, patients show non-specific symptoms or are asymptomatic, which makes it difficult for clinical diagnosis, especially in non-endemic areas. Alternative diagnostic methods that are timely and effective are required to identify infections, particularly in field settings. This article reviews conventional malaria diagnostic methods together with recently developed techniques for both malaria detection and infected erythrocyte separation. Although many alternative techniques have recently been proposed and studied, dielectrophoretic and magnetophoretic approaches are among the promising new techniques due to their high specificity for malaria parasite-infected red blood cells. The two approaches are discussed in detail, including their principles, types, applications and limitations. In addition, other recently developed techniques, such as cell deformability and morphology, are also overviewed in this article.
Malaria is a mosquito borne disease caused by protozoan parasites of the genus Plasmodium , and five species are reported for their infections in humans; namely, Plasmodium falciparum, Plasmodium vivax, Plasmodium malariae, Plasmodium ovale and Plasmodium knowlesi [ 1 , 2 ]. Infection with different Plasmodium species, or sometimes with multiple species, results in different clinical outcomes in patients. The most virulent and fatal species of malaria is P. falciparum , especially when the infection occurs in young children with insufficient protective immunity, and in pregnant women. Although the other Plasmodium species might also cause severe illness in humans, the mortality rate is relatively low.
The 2014 World Health Organization report stated that there were about 584,000 malaria deaths annually worldwide, with 78 % of these deaths occurring in children under 5 years old [ 2 ], largely (>90 %) in Sub-Saharan Africa [ 2 , 3 ]. In Thailand, the Plasmodium species that cause the majority of malaria in humans are P. falciparum (44 %) and P. vivax (47 %), and most cases are reported in regions bordering between Thailand and Myanmar [ 2 – 6 ]. In addition to P. falciparum and P. vivax , another malaria parasite species, P. knowlesi , is emerging as a health issue in Southeast Asia. This zoonotic species possesses the potential for infection of humans, with a natural reservoir in monkeys [ 7 ]. In some areas, for instance in Borneo hospitals of Malaysia, the prevalence of malaria infections by this parasite was as high as 83 % [ 8 ].
Malaria life-cycle
Human-infecting Plasmodium relies on two hosts: humans and certain species of female Anopheles mosquitoes. When an infected mosquito bites a human, sporozoites from the salivary glands of the infected mosquito are injected into the bloodstream and enter the liver of the human, to initiate the exoerythrocytic stage of infection. Within the liver hepatocyte cells, the sporozoites transform and multiply via asexual reproduction to develop into schizonts, structures that can contain thousands of daughter parasites or “merozoites” [ 9 ].
After 6–8 days of development, depending on the Plasmodium species, fully mature schizonts rupture their host hepatocytes to release merozoites into the bloodstream [ 10 ]. The merozoites then invade red blood cells (RBCs) and undergo asexual development within the infected RBC (iRBC), referred to as the erythrocytic cycle. The erythrocytic cycle begins with a tiny ring form, followed by development to a larger amoeboid form termed the trophozoite and finally replication to merozoites within a mature schizont. After rupturing the host’s RBCs, the merozoites invade new RBCs, and the resulting cycles of amplification can result in severe pathology and suffering of the patient due to the burden of iRBC, such as anemia and sequestration of iRBC in deep tissues. Some of the merozoites will develop into male and female gametocytes following RBC invasion, which circulate in the blood and can be ingested by a female mosquito during a blood meal on the infected human. Fertilization between male and female gametocytes occurs in the mosquito midgut to produce zygotes. The zygotes further develop into ookinetes and traverse the mosquito midgut wall to transform into oocysts. Within the oocysts, sporozoites multiply, and the rupture of the mature oocysts release sporozoites that recognize and invade the mosquito salivary glands, where they are ready to continue the life cycle. The development of the malaria parasites in the Anopheles mosquito vector is termed the sporogonic cycle.
After merozoite invasion, the iRBCs undergo parasite-mediated structural transformations, such as remodeling of the iRBC membrane skeleton via exported parasite-encoded proteins. These physical changes include increased rigidity of the iRBC membrane, reduced iRBC deformability and an increased adhesiveness of the iRBCs [ 11 , 12 ]. However, it was recently reported that there was an increased deformability of P. vivax -iRBCs compared to uninfected RBCs (hRBC), which is in contrast to that for P. falciparum -iRBCs [ 13 ]. Furthermore, other physical changes are due to one parasite-specific structure, the haemozoin or malaria pigment. This structure is produced from the haem groups released from the digestion of the haemoglobin within the parasite food vacuole, where the haem groups aggregate into an insoluble crystal [ 14 ].
Due to the difficulty in either detecting malaria-parasite infections, especially in a field setting, or separating infected erythrocytes for a biological study [ 15 – 17 ], many techniques have been developed to meet the specific requirements for each situation. This article aims to review recently developed techniques of malaria diagnosis, both for conventional techniques such as microscopy; rapid diagnostic test (RDT); molecular diagnoses, dominated by PCR-based techniques; and the alternative techniques of dielectrophoretic and magnetophoretic principles, which are based on physical properties of the iRBCs. In particular, the change in the dielectric and magnetic properties of the iRBC due to the released haemozoin has recently been considered as a key bio-marker for malaria diagnosis. Since the importance but difficulty of a correct diagnosis, techniques exploiting other physical and biological properties of iRBCs have also been developed, and some of these will be mentioned in this article.
Conventional techniques
Microscopic diagnosis using blood smears plays an important role in malaria diagnosis because of its ability to diagnose and differentiate each species of malaria, and so it is used as the gold standard for any new detection tool or technique [see, for example, 18 – 22 ]. However, this method still suffers from drawbacks, such as requiring a visual or light microscope with 1000× magnification and relying on skillful and well-trained microscopists. Microscopic diagnosis is a morphology based identification so that Plasmodium species with closely similar in shape or characteristics such as P. knowlesi and P. malariae is prone to fault diagnosis, even by an expert. Recently, some researchers have introduced an image processing technique for microscopy, to avoid human error. However, the main cause of error that is due to a low parasite density was not resolved by this approach [ 23 – 25 ]. This is due to the fact that the average ability of microscopic diagnosis to detect Plasmodium in iRBCs has a threshold of around 10 parasites/µL for a research setting [ 26 ] and in the range of 50–100 parasites/µL for outside a research setting [ 27 ], or less sensitive in a limited resource setting. Fluctuations of parasite density over the course of infection contribute to detection-limit of microscopy-based diagnosis and all other direct detection approaches [ 28 ]. Consequently, direct detection at a single time point has likely resulted in under-estimation of malaria parasite infection rates, especially cases with a low parasite density and asymptomatic malaria at the early and chronic stage of infection. For example, Okell et al. reported that submicroscopic parasite carriage is commonly seen in adult patients in low-endemic settings [ 29 ].
Not only is microscopy laborious and ill-suited for high-throughput use, but in remote rural areas, such as peripheral medical clinics with no electricity and no health-facility resources, microscopy and skillful microscopists are often unavailable. Fortunately, this lack of microscopy in a rural area might be resolved in this near future due to the invention of an origami-based paper microscope [ 30 ]. This device was intentionally developed for malaria control in a poor country.
Another technique, the rapid diagnostic test (RDT), is likely to impact malaria control in the immediate future. A test-kit is able to immunologically detect a number of different malaria antigens such as lactate dehydrogenase (LDH), aldolase and histidine-rich protein-2 (HRP-2) in a small amount of blood (typically 5–15 μL) by a principle similar to that for strip pregnancy test. The detection is performed by an immunochromatographic assay with monoclonal antibodies directed against the target parasite antigen(s) that are impregnated on a test strip. The RDT strip has been extensively tested for its impacts on malaria diagnosis outside research settings for the past few years [ 5 , 20 – 22 , 31 – 35 ], where the implementation of RDTs increased the proportion of patients with a parasite-based diagnosis of malaria compared to microscopy alone, leading to a higher accuracy and timely clinical case management, as well as better cost effectiveness [ 33 – 35 ]. Over 200 RDTs have been currently tested and deployed in the field settings, the most popular ones are, for example, Binax NOW ® , Optimal-IT ® , Paracheck-Pf ® and Paramax-3 [ 36 ]. Some RDTs can detect only single species (either P. falciparum or P. vivax ) while others detect multiple species ( P. falciparum, P. vivax, P. malariae and P. ovale ). Additionally, some RDTs further differentiate between P. falciparum and non- P. falciparum infection, or between specific species. No RDT specifically detects P. knowlesi , although Foster et al. reported that BinaxNOW ® correctly detected non- P. falciparum malaria in P. knowlesi samples but was the least sensitive, detecting only 29 % (8/28; 95 % CI 12–46 %) of fresh samples [ 37 ]. Although this technique is timely and easy to use, it is relatively expensive and prone to false-positive responses due to the persistence of malaria antigens in the blood for up to 2 weeks after the parasite has been cleared from the patient’s circulation. In addition, limits of detection (LOD) of these RDTs rely on an amount of antigen equivalent to 200 iRBCs/µL or 2000–5000 parasites/µL of blood [ 36 ].
The most efficient technique is PCR-based diagnosis which has produced a higher specificity and sensitivity in the identification and differentiation of malaria at the species level. PCR methods can be subdivided into nested PCR, semi-nested PCR, single step multiplex PCR, and real-time or quantitative PCR assays [ 38 , 39 ]. Among them, the simplest and least technically demanding is a loop-mediated isothermal amplification (LAMP) assay [ 40 ]. Generally, PCR is able to detect parasites at a low density, typically below 5 parasites/μL of blood for all five human infecting Plasmodium parasites [ 40 , 41 ]. Recently, it has been reported that saliva, urine and fecal samples of P. falciparum - and P. vivax -infected patients contain malarial DNA that is amplifiable by PCR [ 42 , 43 ]. Although PCR-based diagnosis has a high sensitivity and good specificity, it relies on the complimentary nucleotide sequences between the primer (known sequence) and its counterpart target DNA (unknown sequence), and so parasites with genetically diverse sequences at primer’s target region are prone to detection failure or to a lower amplification efficiency that will reduce the sensitivity of the PCR test. In addition, the sensitivity of detection by PCR-based techniques is affected greatly by copy number of target gene or nucleic acid sequence available in the Plasmodium genome. The most widely used target is small subunit 18S ribosomal RNA gene (18S rDNA). This gene is highly conserved across Plasmodium species and has a moderate copy number-presenting in four to eight copies per parasite. Mitochondrial DNA is another promising target due to it its greater abundance than nuclear DNA (between 30 and 100 copies per parasite). Over the past decade, research efforts have increasingly utilized a well conserved cytochrome b gene in mitochondria genome not only in human Plasmodium [ 44 ], but recently also proven successful in microscopically negative samples in a newly rediscovered Plasmodium species in ungulates [ 45 ]. More PCR-based approaches including wide variety of target DNA or RNA transcripts and their limitations can be seen in recent reviews including Refs [ 10 , 29 , 46 ].
Table 1 shows a comparison of the performance for each diagnostic technique. Currently, PCR has the lowest detection range of the parasite (around 1–5 parasites/µL), but the diagnostic cost is still high, and accessibility is restrictive.
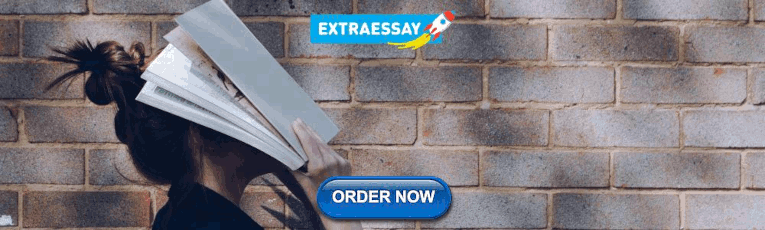
Dielectrophoretic principle
Dielectrophoresis (DEP) was first introduced in 1951 by Pohl [ 59 ]. It is a phenomenon in which polarized neutral particles exposed to a non-uniform electric field are pushed towards or against high electric field intensity regions. The principle of DEP is illustrated in Fig. 1 a–c. A neutral particle exposed to an electric field experiences polarization that causes positive and negative charge accumulation at the opposite sides of the particle. In a uniform electric field, the electrostatic forces are equal on each side of the particle so there is no net force (Fig. 1 a). In contrast, the electrostatic forces are unequal when the particle is exposed to a non-uniform electric field due to the heterogeneous electric field strength experienced across the particle (Fig. 1 b, c). Therefore, the particle experiences a net dielectrophoretic force, which causes particle motion. The direction of the force can be explained in terms of charge accumulation at the interfaces of the particle. In Fig. 1 b, in which a particle is more polarizable than the suspension medium, the dielectrophoretic force drives the particle towards the high electric field intensity region, known as a positive dielectrophoresis (pDEP). When the particle is less polarizable than the medium, the dielectrophoretic force pushes the particle towards the low electric field intensity region (Fig. 1 c), known as a negative dielectrophoresis (nDEP).
Comparison of the net force between different situations: a uniform electric field, b positive dielectrophoresis and c negative dielectrophoresis
At present, DEP has been performed with alternating current (AC), direct current (DC), combined AC/DC and travelling wave, but AC-DEP is the classic DEP that has been used in diverse applications [ 60 – 68 ]. The electrodes used to generate the non-uniform electric field are located inside a microchannel in which the samples are fed (Fig. 2 a). The merit of AC-DEP over the other DEP techniques is its flexibility to manipulate various types of particles due to their frequency-dependent dielectric properties. The direction of the force (pDEP or nDEP), as well as the magnitude, can be controlled by adjusting the signal frequency and electric potential. However, there are a few drawbacks, such as electrode fouling and bubble occurrence inside the channel due to the electrolysis of suspension medium during its operation.
Two methodologies to create a non-uniform electric field: a asymmetric electrode pair and b asymmetric microchannel
Also known as insulator-based DEP (iDEP), DC-DEP utilizes DC applied to electrodes to generate a non-uniform electric field [ 69 – 71 ]. Typically, the electrodes would be located outside a flow channel, while nonconductive insulators are fabricated as a channel wall to create electric-field non-uniformity in a flow passage (Fig. 2 b). This technique helps eliminate the fouling of electrodes as well as the bubbles inside the channel, since there is no contact between the sample medium and electrodes. The main drawback of the DC-DEP approach is the required use of a relatively higher electric potential that might cause a Joule heating problem. The combination of AC/DC-DEP would eliminate the drawbacks of the DC-DEP technique by reducing the electric potential required as well as the Joule heating problem [ 72 – 74 ].
Travelling wave DEP utilizes only AC to manipulate and transport particles [ 75 , 76 ]. Although the mechanism of particle manipulation is similar to that for AC-DEP, there are phase shifts amid the generated electric fields that help serially carrying the particles between each electrode pair.
Although the AC-DEP technique has a few drawbacks, it is still the most practical method for this application. Accordingly, this section will describe the fundamentals of AC-DEP to illustrate the key concepts. The simplified model to derive the time-average AC-DEP expression for a spherical particle is based on the dipole approximation that is given by Eq. ( 1 ) [ 77 ];
where R is the particle radius, ɛ m is the dielectric permittivity of the medium, Re [ f CM ] is the real value of the Clausius–Mossotti factor and E rms is the root mean square of the electric field intensity. The complex Clausius–Mossotti factor is defined by Eq. ( 2 );
where ɛ p and σ p are the dielectric permittivity and electrical conductivity of the particle, ɛ m and σ m are the dielectric permittivity and electrical conductivity of the medium, and ω is the signal frequency. The AC-DEP equation analogous to Eq. ( 1 ) for a multi-pole model has been derived elsewhere. The direction of the force exerting on a particle relies on the real value of the complex Clausius–Mossotti factor ( Re [ f CM ]), which is related to the effective polarizability. The factor depends upon the electrical conductivity and the dielectric permittivity of the particles and the suspension medium, as well as the applied signal frequency. It should be noted that Eq. ( 2 ) can be employed not only for the single-shell model but also for the multi-shell model. However, further modifications for the multi-shell approximation are needed, such as described in Ref. [ 77 ].
In general, it should be understood that each type of biological cell, as well as particle, has its own frequency-dependent characteristic leading to a different magnitude and direction of exerted DEP force in a non-uniform electric field. Therefore, AC-DEP-based micro-devices have been applied for biomedical applications to separate, sort, trap and focus biological cells, such as cancer cells, stem cells and infected cells [ 60 – 62 ], in addition to bacteria, viruses, DNA and proteins [ 63 – 68 ].
With respect to the application for malaria parasite detection, Gascoyne et al. studied the changes in the dielectric properties of iRBCs compared to hRBCs using electrorotation (ROT) and dielectrophoretic crossover approaches [ 78 ]. They found that the dielectrophoretic crossover method was better able to predict the dielectric properties of iRBCs than the ROT approach. A summary of their experimental results [ 62 ] for a spherical shell model is shown in Table 2 . From these results, they explained that the membrane conductivity of iRBCs was higher than that of hRBCs because of RBC membrane barrier deterioration during malaria parasite infection. However, iRBCs retain their internal ions, as shown by their relatively high internal conductivity.
Subsequently, iRBCs were isolated away from hRBCs using two types of electrode configuration (interdigitated and spiral electrodes) [ 62 ]. The system was designed in a manner that the iRBCs experienced a relatively weak nDEP while hRBCs were manipulated under a relatively strong pDEP. The interdigitated electrode was energized by a single-phase sinusoidal signal up to 5 V p-p at a frequency range from 1 to 5 MHz, resulting in the hRBCs being attracted to the electrode edges, while the iRBCs were pushed to the electrode gaps. As a result, iRBCs were swept away by the hydrodynamic force of the suspension medium resulting in a 200-fold concentration of the iRBCs. The other electrode design employed a spiral array of four parallel electrodes. The electrode was activated by a quadrature-phase sinusoidal signal up to 5 V p-p in the frequency range from 1 to 15 MHz, while the second electrode created both traditional AC-DEP and travelling wave. After applying the electric signal, hRBCs were trapped at the electrode edges while iRBCs were levitated and carried towards the center of the electrode, resulting in an approximate 1000-fold enrichment of iRBCs.
In 2004, Gascoyne et al. [ 79 ] proposed a new approach to diagnose malaria, relying on an automated micro-total analysis system (μTAS). The so-called DEP-field-flow-fractionation (DEP-FFF) approach was employed to isolate iRBCs from hRBCs. Simplistically, DEP-FFF is another version of DEP that uses the dielectrophoretic force to position particles in a fluid stream. Different types of particles experience a distinct velocity in accordance with their respective positions due to the parabolic velocity profile of the flow stream (Fig. 3 a). The discrepancy in their velocity causes the particles to emerge at the end of the channel at different times. In the study of Gascoyne et al. [ 79 ], electrode arrays on the channel wall were applied by sinusoidal signals up to 5 V p-p in the frequency range from 40 to 250 kHz and the successful separation of iRBCs from hRBCs by the DEP-FFF approach was obtained. However, the test of integrated flow-through PCR for malaria diagnosis was not included in that report.
Two applications of the dielectrophoretic force: a cell separation and b cell characterization
Recently, AC-DEP was used not only to discriminate early stage iRBCs from hRBCs but also used to predict cellular mechanical properties [ 50 ]. In this study, both iRBCs and hRBCs were trapped at the electrode edges when the electrodes were activated. The iRBCs were able to retain their original shape at the edges due to their greater stiffness and weaker DEP force. In contrast, the hRBCs were significantly deformed due to their stronger pDEP effect. The noticeable deformation of hRBCs over iRBCs offered the possibility of a visible and mechanical discrimination (Fig. 3 b). In addition, the cell deformability could be measured by estimating the DEP force exerted on the hRBCs from Eq. ( 1 ) and the stretch ratio.
The AC-DEP method exploits dissimilar dielectric properties between iRBCs and hRBCs for cell discrimination and might be the most appropriate of the different DEP techniques for malaria parasite detection. However, due to the low difference in, and the heterogeneity of, the electrical properties and diameter of iRBCs and hRBCs, the employment of the dielectrophoretic approach is quite limited in malaria parasite research.
Magnetophoretic principle
With respect to the life cycle of the malaria parasite, after each sporozoite invades a liver cell and undergoes asexual development followed by hepatocyte lysis, the resulting merozoites are swept into the bloodstream to infect RBCs. The parasite digests haemoglobin leaving haem groups that aggregate into an insoluble brown pigment haemozoin. The number and size of haemozoin pigments depends on the developmental stage of the parasite [ 80 , 81 ]. Accordingly, the level of haemozoin in RBCs has become a key physical property that is used to detect or separate malaria parasite infected RBCs. Studies using spectroscopic and crystallographic techniques revealed that haemozoin has a magnetic structure that is a synthetic biomineral, specifically, beta-haematin [ 82 ]. The molecular structure and composition of beta-haematin is generally a single domain crystal of magnetite (Fe 3 O 4 ) or greigie (Fe 3 S 4 ) [ 83 , 84 ]. Additionally, its magnetic properties have been determined using electron paramagnetic resonance and Moessbauer spectroscopy, and the results revealed that haemozoin has a Fe 3+ component [ 85 ]. The magnetic properties of iRBCs were quantified, where the magnetophoretic mobility and net volume magnetic susceptibility of iRBCs were found to be 2.94 × 10 −6 mm 3 s/kg and 1.80 × 10 −6 (relative to water), respectively, at a haemoglobin to haemozoin fraction of 0.5 [ 80 , 86 ].
When a magnetophoretic force acts on a particle that lies in a non-uniform magnetic field, the magnitude of the magnetophoretic force is given by Eq. ( 3 );
where μ medium is the magnetic permeability of the suspension medium (N/A 2 ), μ particle is the magnetic permeability of the particles (N/A 2 ), a is the radius of the particles and H is the magnetic field strength (A/m). Considering Eq. ( 3 ), the magnitude of magnetophoretic force is proportional to the cubic size of the particles and also the gradient of magnetic field. Thus, Eq. ( 3 ) can be simplified to that of Eq. ( 4 );
where µ 0 is the permeability of free space (4π × 10 −7 N/A 2 ) and \(\Delta \chi\) is the relative magnetic susceptibility of particles to suspension medium ( χ target − χ buffer ). From Eq. ( 4 ), the magnitude of the magnetophoretic force is proportional to the relative magnetic susceptibility. The magnetophoretic principle for malaria parasite detection then takes advantage of the significantly different magnetic properties between hRBCs and iRBCs, as shown in Table 3 .
Paul et al. were probably the first research group to apply a magnetophoretic force for separating iRBCs in practice [ 52 , 87 ], in which they used a fluid channel containing two chambers of dimensions of 25 × 10 × 52 mm 3 (first chamber) and 13 × 13 × 9 mm 3 (second chamber). Both chambers were filled with 25-µm diameter stainless wire at 4.6 and 7.6 % density for the first and second chamber, respectively. The channel was placed between a 0.7 T permanent magnet, as shown in Fig. 4 a. They reported that about 75 % of iRBCs in the trophozoite and schizont stages were immobilized in the chamber under an operating flow velocity of 0.19 mm/s. A similar idea was later applied by employing a 0.7 T yoke-shaped neodymium magnet of 50 × 30 × 12 mm 3 with a microchannel between the dipole magnets [ 54 ]. The channel contained stainless steel wool with a size range from 30 to 50 µm diameter and was flushed with isotonic sucrose solution containing 0.75 % (w/v) gelatin for the concentration stage and 0.2 % (v/v) RPMI 1640 and 1 % (w/v) BSA for the subsequent depletion stage. The device could increase the concentration of iRBCs up to 96 %, where most of the trapped iRBCs were in the schizont stage due to their high magnetic susceptibility. It should be noted here that some types of stainless steel wool could help inducing a local magnetic field gradient as well as magnetophoretic force, depending on their composition.
Applications of magnetophoresis: a locally strengthening the force using metal wool, b switching the magnetic field direction using opposing poles, c creating the non-homogenous field using a ferromagnetic wire and d manipulating cells to a location under high magnetic force using a centrifugal force
Subsequently, Zimmerman et al. [ 53 ] used two ferrite permanent magnets separated by 1.27 mm to trap iRBCs inside a microchannel. The magnetic field strength at the midline between the surfaces of the two permanent magnets was 1.1 × 10 6 A/m. At this position, where the strongest magnetic field gradient was induced, iRBCs would be forced to move towards the gap between the two magnets perpendicularly to the mainstream of blood flow in the channel (Fig. 4 b). At a velocity of 1.2 mm/s, the concentration of iRBCs was increased 40-, 250-, 250- and 375-fold for P. falciparum , P. vivax , P. malariae and P. ovale , respectively.
Another approach for malaria diagnosis is to utilize the changes in both the magnetic and optical properties of the iRBCs, since they exhibit a high magnetic anisotropy and have optical dichroism properties. Based on this principle, an iRBCs detection technique was developed using the magneto-optic principle, which allowed a high-sensitivity detection of the malaria pigment [ 88 , 89 ].
An additional approach for the magnetophoretic principle is the employment of magnetic nanoparticles as label markers [ 55 ]. The addition of magnetic nanoparticles to attach a magnetic crystal of β-haematin, which can amplify the Raman spectroscopy signal, allowed the detection of iRBCs at an early stage. The other approach is to separate the iRBCs under a continuous flow in a microfluidics channel. For this system, an applied uniform 0.6 T magnetic field across a 100-µm diameter nickel wire along the channel (Fig. 4 c) was reported [ 56 ]. The ferromagnetic wire increased the magnetic field gradient resulting in a more easily controlled lateral movement of the iRBCs away from the mainstream of blood flow. Overall, a separation efficiency of almost 100 % was obtained at a flow rate of <0.14 μL/min.
Recently, the magnetophoretic force has been combined with other physical properties, such as cell rigidity, to detect or separate iRBCs [ 57 , 58 ]. A schematic illustration of one such device is shown in Fig. 4 d. After the blood sample flows through a narrow channel of 50 μm width and 25 mm length, the eluted blood was centrifuged with an accumulation of iRBCs relatively close to the wall of the microchannel. The channel was then put in a micromagnetic resonance relaxometry (MRR) receiver coil with a 0.5 T permanent magnet, where the presence of iRBCs in the blood sample was detected and the signal transmitted through the MRR spectrometer.
These studies using the magnetophoretic principle employed an external magnetic field, where it was found that the detection or separation performance could be enhanced, but to a limited extent. This was due to the fact that the system could not provide a sufficiently high magnetic-force locally to effectively and precisely manipulate iRBCs. To resolve this issue, a system providing a high magnetic field gradient would be a key component in generating a high magnetophoretic force [ 90 ]. One promising technique is the addition of a ferromagnetic substance into the system. The substance can modify the magnetic field strength profile in the area around it. However, the gradient of magnetic field resulting from this ferromagnetism happens only in a tiny area around the ferromagnetic substance. Considering this factor, the employment of a microfluidics system would be an appropriate choice for malaria parasite detection. Furthermore, a high magnetic field gradient can be generated by various other factors, such as the type of ferromagnetic material, the orientation of the component and the direction of the uniform external magnetic field.
Recent trends
From the literature, many studies take advantage of the existence of iRBC-specific haemozoin, which is a prominent biomarker for malaria diagnosis without sophisticated sample preparation. However, the detection or separation-based on haemozoin alone might have several pitfalls causing low efficiency of diagnosis. For example, electrical properties (see in Table 2 ) of uninfected and infected red blood cells are not significantly different; therefore, a separation of iRBCs with dielectrophoretic force alone may be impractical. Moreover, iRBCs at the ring stage contain low concentration or non-existent haem crystal [ 91 ]. These factors might have been responsible for the low efficiency haemozoin-based detection and separation of early stage infections in several past studies.
On the contrary, one of outstanding capabilities of dielectrophoresis and magnetophoresis (see in Table 1 ) is its ability to enrich the concentration of infected erythrocytes. The enrichment and separation without destroying of the parasite, which could not be obtained from microscopy, RDTs and PCR techniques, might be invaluable in malaria parasite research. Additionally, because of the portability, minimal expertise and ease in use, methods based upon dielectrophoretic and magnetophoretic principles could be considered as an alternative for malaria diagnosis.
In the last few years several research groups have tried to employ other new techniques to enhance the detection or separation of iRBCs. One of these approaches is the use of non-woven fabric (NWF) size-based filtration, which is simple and cheap [ 92 ]. The prototype single-use NWF filter demonstrated the successful removal of 99 % leukocytes from 5 mL of malaria-infected blood, which helped reducing the white blood cells contamination of iRBCs during a biological study. Another study found that the morphological changes on the surface of the malaria-iRBCs could be a biomarker for malaria diagnosis [ 93 ].
In addition to the physical change in RBCs following malaria infection, the reduced deformability of iRBCs is a property that could be employed for malaria detection and separation, at least for P. falciparum -iRBCs. Moreover, it has been reported that the iRBC deformability could be used to sort P. falciparum -iRBCs at different developmental stages and to enrich the ring-stage by 2500-fold [ 94 ]. Several other works have studied the relationship between the iRBC deformability and developmental stage of the infecting Plasmodium [ 95 , 96 ] and the possibilities of a deformability-based technique to sort iRBCs [ 97 – 100 ].
More recent research reported that the high optical absorbance and nanosize of haemozoin could generate a transient vapor nanobubble around haemozoin when exposed to a short NIR picosecond laser pulse. The acoustic signals of these induced nanobubbles provided a transdermal, non-invasive and rapid detection of malaria infection in animals without using any reagents or drawing blood [ 101 ].
Several studies have employed a new platform to an existing conventional principle. For example, flow cytometry using standard nucleic acid-based staining methods was employed for a rapid and highly sensitive detection of iRBCs [ 102 , 103 ], while a paper-based 2D platform that enabled multistep assays was also developed to detect malaria antigens in a low-resource setting [ 104 ]. A droplet microfluidics platform was employed for highly sensitive and quantitative detection of iRBCs based on an enzyme activity measurement [ 105 ]. In addition, a combination of dielectrophoretic and magnetophoretic forces has also been employed for the separation of iRBCs [ 106 ].
Conclusions
Globally, around 3.2 billion people are estimated to be at risk of being infected and developing malaria, and 1.2 billion people are at a high risk. According to the latest estimation, over 200 million cases of malaria occur around the world annually, and the disease leads to over 500,000 deaths each year. Early detection could save lives and prevent disease outbreaks, as well as allow parasite enrichment for biology studies, which are important topics in malaria research. The conventional microscopic examination of blood smears, antigen-based rapid test and molecular biology-based diagnosis all have some limitations for effective employment in low-resource setting areas. Therefore, researchers around the world are looking for new options. Among various techniques, dielectrophoretic and magnetophoretic principles have recently became attractive possibilities for malaria diagnosis due to the unique changes in the electrical and magnetic properties of iRBCs compared to hRBCs. Moreover, alternative techniques, such as cell morphology and deformability, have been proposed to be suitable bio-markers for the future. Not only is a knowledge of parasite biological properties critical, but the development of an engineering system is also an important factor, and should be studied in parallel. One example is a magnetic-field generating system that could create a high magnetic field gradient over a large area. Another solution might be a combination of two or three detection techniques, which might be able to increase the specificity to iRBCs and so enhance the ability for malaria detection or infected erythrocyte separation.
Shapiro HM, Apte SH, Chojnowski GM, Hänscheid T, Rebelo M, Grimberg BT. Cytometry in malaria—a practical replacement for microscopy? Curr Protoc Cytom. 2013;chapt 11:Unit 11.20 doi: 10.1002/0471142956.cy1120s65 .
WHO. World Malaria Report. Geneva: World Health Organization; 2014.
Google Scholar
Gething PW, Elyazar IRF, Moyes CL, Smith DL, Battle KE, Guerra CA, et al. A long neglected world malaria map: Plasmodium vivax endemicity in 2010. PLoS Negl Trop Dis. 2012;6:1814.
Article Google Scholar
Coleman RE, Sattabongkot J, Promstaporm S, Maneechai N, Tippayachai B, Kengluecha A, et al. Comparison of PCR and microscopy for the detection of asymptomatic malaria in a Plasmodium falciparum/vivax epidemic area in Thailand. Malar J. 2006;14:121.
Sudhinaraset M, Briegleb C, Aung M, Khin H, Aung T. Motivation and challenges for use of malaria rapid diagnostic tests among informal providers in Myanmar: a qualitative study. Malar J. 2015;14:61.
Article PubMed PubMed Central Google Scholar
Baum E, Sattabongkot J, Sirichaisinthop J, Kiattibutr K, Davies DH, Jain A, et al. Submicroscopic and asymptomatic Plasmodium falciparum and Plasmodium vivax infections are common in western Thailand—molecular and serological evidence. Malar J. 2015;14:95.
WHO. Malaria. Geneva: World Health Organization. 2015. http://www.who.int/ith/diseases/malaria/en/ . Accessed 20 May 2015.
Cox-Singh J, Davis TM, Lee KS, Shamsul SS, Matusop A, Ratnam S. Plasmodium knowlesi malaria in humans is widely distributed and potentially life threatening. Clin Infect Dis. 2008;46:165–71.
Article CAS PubMed PubMed Central Google Scholar
Tarun AS, Baer K, Dumpit RF, Gray S, Lejarcegui N, Frevert U, et al. Quantitative isolation and in vivo imaging of malaria parasite liver stages. Int J Parasitol. 2006;36:1283–93.
Article CAS PubMed Google Scholar
Bousema T, Okell L, Felger I, Drakeley C. Asymptomatic malaria infections: detectability, transmissibility and public health relevance. Nat Rev Microbiol. 2014;12:833–40.
Cooke BM, Mohandas N, Coppel RL. The malaria-infected red blood cell: structural and functional changes. Adv Parasitol. 2001;50:1–86.
Xu X, Efremov AK, Li A, Lai L, Dao M, Lim CK, et al. Probing the cytoadherence of malaria infected red blood cells under flow. PLoS One. 2013;8:e64763.
Handayani S, Chiu DT, Tjitra E, Kuo JS, Lampah D, Kenangalem E, et al. High deformability of Plasmodium vivax -infected red blood cells under microfluidic conditions. J Infect Dis. 2008;199:445–50.
Pagola S, Stephens PW, Bohle DS, Kosar AD, Madsen SK. The structure of malaria pigment β-haematin. Nature. 2000;404:307–10.
Trager W, Jensen JB. Continuous culture of Plasmodium falciparum : its impact on malaria research. Int J Parasitol. 1997;27:989–1006.
Druilhe P, Mazier D, Brandicourt O, Gentilini M. One-step Plasmodium falciparum cultivation—application to in vitro drug testing. Tropenmed Parasitol. 1983;34:233–4.
CAS PubMed Google Scholar
LeRoux M, Lakshmanan V, Daily JP. Plasmodium falciparum biology: analysis on in vitro versus in vivo growth conditions. Trends Parasitol. 2009;25:474–81.
Article PubMed Google Scholar
Wongsrichanalai C, Barcus MJ, Muth S, Sutamihardja A, Wernsdorfer WH. A review of malaria diagnostic tools: microscopy and rapid diagnostic test (RDT). Am J Trop Med Hyg. 2007;77:119–27.
PubMed Google Scholar
Tangpukdee N, Duangdee C, Wilairatana P, Krudsiid S. Malaria diagnosis: a brief review. Korean J Parasitol. 2009;47:93–102.
Alam MS, Mohon AN, Mustafa S, Khan WA, Islam N, Karim MJ, et al. Real-time PCR assay and rapid diagnostic tests for the diagnosis of clinically suspected malaria patients in Bangladesh. Malar J. 2011;10:175.
Ouattara A, Doumbo S, Saye R, Beavogui AH, Traorè B, Djimdè A, et al. Use of a pLDH-based dipstick in the diagnostic and therapeutic follow-up of malaria patients in Mali. Malar J. 2011;10:345.
Batwala V, Magnussen P, Nuwaha F. Comparative feasibility of implementing rapid diagnostic test and microscopy for parasitological diagnosis of malaria in Uganda. Malar J. 2011;10:373.
Díaz G, González FA, Remero E. A semi-automatic method for quantification and classification of erythrocytes infected with malaria parasites in microscopic images. J Biomed Inform. 2009;42:296–307.
Tek FB, Dempster AG, Kale I. Computer vision for microscopy diagnosis of malaria. Malar J. 2009;8:153.
Das DK, Maiti AK, Chakraborty C. Automated system for characterization and classification of malaria-infected stages using light microscopic images of thin blood smears. J Microsc. 2015;257:238–52.
McNamara DT, Kasehagen L, Grimberg B, Cole-Tobian JL, Collins WE, Zimmerman PA. Diagnosing infection levels of four human malaria parasite species by a polymerase chain reaction/ligase detection reaction fluorescent microsphere-based assay. Am J Trop Med Hyg. 2006;74:413–21.
CAS PubMed PubMed Central Google Scholar
Payne D. Use and limitations of light microscopy for diagnosing malaria at the primary health care level. Bull World Health Organ. 1988;66:621–8.
Hawkins K, Burton R, LaBarre P. Diagnostics to support malaria elimination: choosing an appropriate biomarker to target the subclinical Plasmodium falciparum transmission reservoir. In: IEEE 2014 global humanitarian technology conference; 2014 October 10–13. San Jose: IEEE; 2014.
Okell LC, Bousema T, Griffin JT, Ouédraogo AL, Ghani AC, Drakeley CJ. Factors determining the occurrence of submicroscopic malaria infections and their relevance for control. Nat Commun. 2012;3:1237.
Cybulski J, Clements J, Prakash M. Foldscope: origami-based paper microscope. Short report. Stanford: Stanford University; 2014.
Masanja IM, McMorrow ML, Maganga MB, Sumari D, Udhayakumar V, McElroy PD, et al. Quality assurance of malaria rapid diagnostic tests used for routine patient care in rural Tanzania: microscopy versus real-time polymerase chain reaction. Malar J. 2015;14:85.
Fransisca L, Kusnanto JH, Satoto TBT, Sebayang B, Supriyanto Andriyan E, et al. Comparison of rapid diagnostic test Plasmotec Malaria-3, microscopy, and quantitative real-time PCR for diagnoses of Plasmodium falciparum and Plasmodium vivax infections in Mimika Regency, Papua, Indonesia. Malar J. 2015;14:103.
Hansen KS, Grieve E, Mikhail A, Mayan I, Mohammed N, Anwar M, et al. Cost-effectiveness of malaria diagnosis using rapid diagnostic tests compared to microscopy or clinical symptoms alone in Afghanistan. Malar J. 2015;14:217.
Silumbe K, Yukich JO, Hamainza B, Bennett A, Earle D, Kamuliwo M, et al. Costs and cost-effectiveness of a large-scale mass testing and treatment intervention for malaria in Southern Province, Zambia. Malar J. 2015;14:211.
Boyce RM, Muiru A, Reyes R, Ntaro M, Mulogo E, Matte M, et al. Impact of rapid diagnostic tests for the diagnosis and treatment of malaria at a peripheral health facility in Western Uganda: an interrupted time series analysis. Malar J. 2015;14:203.
WHO. Malaria rapid diagnostic test performance: results of WHO product testing of malaria RDTs: round 5 (2013). Geneva: World Health Organization; 2014.
Foster D, Cox-Singh J, Mohamad DS, Krishna S, Chin PP, Singh B. Evaluation of three rapid diagnostic tests for the detection of human infections with Plasmodium knowlesi . Malar J. 2014;13:60.
Ongagna-Yhombi SY, Corstjens P, Geva E, Abrams WR, Barber CA, Malamud D, et al. Improved assay to detect Plasmodium falciparum using an uninterrupted, semi-nested PCR and quantitative lateral flow analysis. Malar J. 2013;12:74.
Lee PC, Chong ET, Anderios F, Lim YA, Chew CH, Chua KH. Molecular detection of human Plasmodium species in Sabah using PlasmoNex™ multiplex PCR and hydrolysis probes real-time PCR. Malar J. 2015;14:28.
Cook J, Aydin-Schmidt B, González IJ, Bell D, Edlund E, Nassor MH, et al. Loop-mediated isothermal amplification (LAMP) for point-of-care detection of asymptomatic low-density malaria parasite carriers in Zanzibar. Malar J. 2015;14:43.
Moody A. Rapid diagnostic tests for malaria parasites. Clin Microbiol Rev. 2002;15:66–78.
Putaporntip C, Buppan P, Jongwutiwes S. Improved performance with saliva and urine as alternative DNA sources for malaria diagnosis by mitochondrial DNA-based PCR assays. Clin Microbiol Infect. 2011;17:1484–91.
Jirku M, Pomajbíková K, Petrželková KJ, Huzová Z, Modrý D, Lukeš J. Detection of Plasmodium spp. in human feces. Emerg Infect Dis. 2012;18:634–6.
Steenkeste N, Incardona S, Chy S, Duval L, Ekala MT, Lim P. Towards high-throughput molecular detection of Plasmodium : new approaches and molecular markers. Malar J. 2009;8:86.
Templeton TJ, Asada M, Jiratanh M, Ishikawa SA, Tiawsirisup S, Sivakumar T, et al. Ungulate malaria parasites. Sci Rep. 2016;6:23230. doi: 10.1038/srep23230 .
Zimmerman PA, Howes RE. Malaria diagnosis for malaria elimination. Curr Opin Infect Dis. 2015;28:446–54. doi: 10.1097/QCO.0000000000000191 .
Murray CK, Gasser RA, Magill AJ, Miller RS. Update on rapid diagnostic testing for malaria. Clin Microbiol Rev. 2008;21:97–110.
Hendriksen ICE, Mtove G, Pedro AJ, Gomes E, Silamut K, Lee SJ, et al. Evaluation of a PfHRP 2 and a pLDH-based rapid diagnostic test for the diagnosis of severe malaria in 2 populations of African children. Clin Infect Dis. 2011;52:1100–7.
Khan SA, Ahmed S, Mushahid N, Anwer M, Shahzad S, Khan FA, et al. Comparison of real time polymerase chain reaction with microscopy and antigen detection assay for the diagnosis of malaria. J Coll Physicians Surg Pak. 2013;23:787–92.
Du E, Dao M, Suresh S. Quantitative biomechanics of healthy and diseased human red blood cells using dielectrophoresis in a microfluidic system. Extreme Mech Lett. 2014;1:35–41.
Heidelberger M, Mayer MM, Demarest CR. Studies in human malaria: I. The preparation of vaccines and suspensions containing plasmodia. J Immunol. 1946;52:325–30.
Paul F, Roath S, Melville D. Separation of malaria-infected erythrocytes from whole blood: use of a selective high-gradient magnetic separation technique. Lancet. 1981;2:70–1.
Zimmerman PA, Thomson JM, Fujioka H, Collins WE, Zborowski M. Diagnosis of malaria by magnetic deposition microscopy. Am J Trop Med Hyg. 2006;74:568–72.
PubMed PubMed Central Google Scholar
Bhakdi SC, Ottinger A, Somsri S, Sratongno P, Pannadaporn P, Chimma P, et al. Optimized high gradient magnetic separation for isolation of Plasmodium -infected red blood cells. Malar J. 2010;9:38.
Yuen C, Liu Q. Magnetic field enriched surface enhanced resonance Raman spectroscopy for early malaria diagnosis. J Biomed Opt. 2012;17:017005.
Nam J, Huang H, Lim H, Lim C, Shin S. Magnetic separation of malaria-infected red blood cells in various developmental stages. Anal Chem. 2013;85:7316–23.
Kong TF, Ye W, Peng WK, Hou HW, Marcos, Preiser PR, et al. Enhancing malaria diagnosis through microfluidic cell enrichment and magnetic resonance relaxometry detection. Sci Rep. 2015;5:11425.
Peng WK, Kong TF, Ng CS, Chen L, Huang Y, Bhagat AAS, et al. Micromagnetic resonance relaxometry for rapid label-free malaria diagnosis. Nat Med. 2014;20:1069–73.
Pohl HA. The motion and precipitation of suspensoids in divergent electric fields. J Appl Phys. 1951;22:869–71.
Article CAS Google Scholar
Alshareef M, Metrakos N, Juarez Perez E, Azer F, Yang F, Yang X. Separation of tumor cells with dielectrophoresis-based microfluidic chip. Biomicrofluidics. 2013;7:803.
Prieto JL, Nourse J, Lu J, Flanagan L, Lee AP. Dielectrophoretic separation of heterogeneous stem cell populations. In: 14th international conference on miniaturized systems for chemistry and life sciences (MicroTAS 2010). The Netherlands; 2010. p.890–2.
Gascoyne P, Mahidol C, Ruchirawat M, Satayavivad J, Watcharasit P, Becker F. Microsample preparation by dielectrophoresis: isolation of malaria. Lab Chip. 2002;2:70–5.
Bisceglia E, Cubizolles M, Trainito CI, Berthier J, Pudda C, Français O. A generic and label free method based on dielectrophoresis for the continuous separation of microorganism from whole blood samples. Sens Actuators B Chem. 2015;212:335–43.
Hamada R, Takayama H, Shonishi Y, Mao L, Nakano M, Suehiro J. A rapid bacteria detection technique utilizing impedance measurement combined with positive and negative dielectrophoresis. Sens Actuators B Chem. 2013;181:439–45.
Sonnenberg A, Marciniak JY, McCanna J, Krishnan R, Rassenti L, Kipps TJ. Dielectrophoretic isolation and detection of cfc-DNA nanoparticulate biomarkers and virus from blood. Electrophoresis. 2013;34:1076–84.
Asbury CL, Diercks AH, van den Engh G. Trapping of DNA by dielectrophoresis. Electrophoresis. 2002;23:2658–66.
Regtmeier J, Duong TT, Eichhorn R, Anselmetti D, Ros A. Dielectrophoretic manipulation of DNA: separation and polarizability. Anal Chem. 2007;79:3925–32.
Chen H, Liu Y, Zhang H, Yu L, Zhu Y, Li D. Separation and manipulation of rare-earth oxide particles by dielectrophoresis. Chin J Chem Eng. 2010;18:1034–7.
Lapizco-Encinas BH, Simmons BA, Cummings EB, Fintschenko Y. Dielectrophoretic concentration and separation of live and dead bacteria in an array of insulators. Anal Chem. 2004;76:1571–9.
Kang KH, Kang Y, Xuan X, Li D. Continuous separation of microparticles by size with direct current-dielectrophoresis. Electrophoresis. 2006;27:694–702.
Kang Y, Li D, Kalams SA, Eid JE. DC-dielectrophoretic separation of biological cells by size. Biomed Microdevices. 2008;10:243–9.
Viefhues M, Wegener S, Rischmuller A, Schleef M, Anselmetti D. Dielectrophoresis based continuous-flow nano sorter: fast quality control of gene vaccines. Lab Chip. 2013;13:3111–8.
Zhu J, Xuan X. Dielectrophoretic focusing of particles in a microchannel constriction using DC-biased AC flectric fields. Electrophoresis. 2009;30:2668–75.
Zellner P, Shake T, Hosseini Y, Nakidde D, Riquelme MV, Sahari A. 3D insulator-based dielectrophoresis using DC-biased, AC electric fields for selective bacterial trapping. Electrophoresis. 2015;36:277–83.
Cheng IF, Froude VE, Zhu Y, Chang HC. A continuous high-throughput bioparticle sorter based on 3D traveling-wave dielectrophoresis. Lab Chip. 2009;9:3193–201.
van den Driesche S, Rao V, Puchberger-Enengl D, Witarski W, Vellekoop MJ. Continuous cell from cell separation by traveling wave dielectrophoresis. Sens Actuators B Chem. 2012;170:207–14.
Jones TB. Electromechanics of particles. Cambridge: Cambridge University Press; 1995.
Book Google Scholar
Gascoyne P, Pethig R, Satayavivad J, Becker FF, Ruchirawat M. Dielectrophoretic detection of changes in erythrocyte membranes following malarial infection. Biochim Biophys Acta. 1997;1323:240–52.
Gascoyne P, Satayavivad J, Ruchirawat M. Microfluidic approaches to malaria detection. Acta Trop. 2004;89:357–69.
Moore LR, Fujioka H, Williams PS, Chalmers JJ, Grimberg B, Zimmerman PA, et al. Hemoglobin degradation in malaria-infected erythrocytes determined from live cell magnetophoresis. FASEB J. 2006;20:747–9.
Egan TJ. Recent advances in understanding the mechanism of hemozoin (malaria pigment) formation. J Inorg Biochem. 2007;102:1288–99.
Egan TJ. Physico-chemical aspects of hemozoin (malaria pigment) structure and formation. J Inorg Biochem. 2002;91:19–26.
Dunin-Borkowski RE, McCartney MR, Frankel RB, Bazylinski DA, Posfai M, Buseck PR. Magnetic microstructure of magnetotactic bacteria by electron holography. Science. 1998;282:1868–70.
Bazylinski DA, Frankel RB. Magnetosome formation in prokaryotes. Nat Rev Microbiol. 2004;2:217–30.
Bohle DS, Debrunner P, Jordan PA, Madsen SK, Schulz CE. Aggregated heme detoxification byproducts in malarial trophozoites: beta-hematin and malaria pigment have a single S = 5/2 iron environment in the bulk phase as determined by EPR and magnetic Moessbauer spectroscopy. J Am Chem Soc. 1998;120:8255–6.
Zborowski M, Ostera GR, Moore LR, Milliron S, Chalmers JJ, Schechter AN. Red blood cell magnetophoresis. Biophys J. 2003;84:2638–45.
Fairlamb AH, Paul F, Warhurst DC. A simple magnetic method for the purification of malarial pigment. Mol Biochem Parasitol. 1984;12:307–12.
Newman DM, Heptinstall J, Matelon RJ, Luke S, Wears ML, Beddow J, et al. A magneto-optic route toward the in vivo diagnosis of malaria: preliminary results and preclinical trial data. Biophys J. 2008;95:994–1000.
Orbán A, Butykai A, Molnár A, Pröhle Z, Fulop G, Zelles T, et al. Evaluation of a novel magneto-optical method for the detection of malaria parasites. PLoS One. 2014;9:e96981.
Karl S, Woodward RC, Davis TME, St. Pierre TG. Manufacture and testing of a high field gradient magnetic fractionation system for quantitative detection of Plasmodium falciparum gametocytes. In: 8th international conference on the scientific and clinical applications of magnetic carriers (AIP); 2010. p. 135–40.
Delahunt C, Horning MP, Wilson BK, Proctor J, Hegg MC. Limitations of haemozoin-based diagnosis of Plasmodium falciparum using dark-field microscopy. Malar J. 2014;13:147.
Tao ZY, Xia H, Cao J, Gao Q. Development and evaluation of a prototype non-woven fabric filter for purification of malaria-infected blood. Malar J. 2011;10:251.
Hsu YH, Lu P, Coleman JL, Tang WC. A microfluidic platform to isolate avian erythrocytes infected with Plasmodium gallinaceum malaria parasites based on surface morphological changes. Biomed Microdevices. 2011;13:995–1004.
Guo Q, Matthews K, Deng X, Duffy S, Ma H. Deformability-based sorting of red blood cells to enrich for parasitized cells in Falciparum malaria. In: 19th international conference on miniaturized systems for chemistry and life sciences (MicroTAS 2015). Korea; 2015. p. 370–2.
Nukaga M, Kim SH, Yahata K, Fujii T, Kaneko O, Kimura H. On-Chip deformability measurement of malaria parasite-infected red blood cells by dielectrophoretic force. In: 19th international conference on miniaturized systems for chemistry and life sciences (MicroTAS 2015). Korea; 2015. p. 484–6.
Santoso AT, Deng X, Lee JH, Matthews K, Islamzada E, McFaul S, et al. Red blood cell-phoresis enabling high-throughput deformability analysis of malaria parasitism. In: 19th international conference on miniaturized systems for chemistry and life sciences (MicroTAS 2015). Korea; 2015. p. 97–9.
Song H, Hashash YMA. Characterization of stress-strain behavior of red blood cells (RBCs), part II: response of malaria-infected RBCs. Inverse Probl Sci Eng. 2015;23:413–24.
Krüger T, Holmes D, Coveney PV. Deformability-based red blood cell separation in deterministic lateral displacement devices—a simulation study. Biomicrofluidics. 2014;8:054114.
Wu T, Feng JJ. Simulation of malaria-infected red blood cells in microfluidic channels: passage and blockage. Biomicrofluidics. 2013;7:044115.
Article PubMed Central Google Scholar
Ye T, Phan-Thien N, Khoo BC, Lim CT. Stretching and relaxation of malaria-infected red blood cells. Biophys J. 2013;105:1103–9.
Lukianova-Hleb EY, Campbell KM, Constantinou PE, Braam J, Olson JS, Ware RE, et al. Hemozoin-generated vapor nanobubbles for transdermal reagent- and needle-free detection of malaria. Proc Natl Acad Sci USA. 2014;11:900–5.
Roobsoong W, Maher SP, Rachaphaew N, Barnes SJ, Williamson KC, Sattabongkot J, et al. A rapid sensitive, flow cytometry-based method for the detection of Plasmodium vivax -infected blood cells. Malar J. 2014;13:55.
Vorobjev IA, Buchholz K, Prabhat P, Ketman K, Egan ES, Marti M, et al. Optimization of flow cytometric detection and cell sorting of transgenic Plasmodium parasites using interchangeable optical filters. Malar J. 2012;11:312.
Fu E, Liang T, Spicar-Mihalic P, Houghtaling J, Ramachandran S, Yager P. Two-Dimensional paper network format that enables simple multistep assays for use in low-resource settings in the context of malaria antigen detection. Anal Chem. 2012;84:4574–9.
Juul S, Nielsen C, Labouriau R, Roy A, Tesauro C, Jensen PW, et al. Droplet microfluidics platform for highly sensitive and quantitative detection of malaria-causing Plasmodium parasites based on enzyme activity measurement. ACS Nano. 2012;6:10676–83.
Buranapong J, Pimpin A, Srituravanich W, Suzuki Y. A microfluidic chip coupled with magnetophoretic and dielectrophoretic forces for separating malaria-infected red blood cells. In: 19th international conference on miniaturized systems for chemistry and life sciences (MicroTAS 2015). Korea; 2015. p. 382–4.
Download references
Authors’ contributions
SK, JB and AP conceived the idea, organized the structure of the manuscript, performed the searches for related previous work and collected the data. The manuscript was drafted by SK, JB, WS and AP. MK reorganized and provided updated biological data. All authors read and approved the final manuscript.
Acknowledgements
This work received financial support from Chulalongkorn University through the Chulalongkorn Academic Advancement into Its second Century Project (Smart Medical Device). The authors sincerely thank Thomas Templeton for his English proof reading.
Competing interests
The authors declare that they have no competing interests.
Author information
Authors and affiliations.
Department of Mechanical Engineering, Faculty of Engineering, Chulalongkorn University, Bangkok, 10330, Thailand
Surasak Kasetsirikul, Jirayut Buranapong, Werayut Srituravanich & Alongkorn Pimpin
Animal Vector-Borne Diseases Research Group, The Veterinary Parasitology Unit, Department of Pathology, Faculty of Veterinary Science, Chulalongkorn University, Bangkok, 10330, Thailand
Morakot Kaewthamasorn
You can also search for this author in PubMed Google Scholar
Corresponding author
Correspondence to Alongkorn Pimpin .
Rights and permissions
Open Access This article is distributed under the terms of the Creative Commons Attribution 4.0 International License ( http://creativecommons.org/licenses/by/4.0/ ), which permits unrestricted use, distribution, and reproduction in any medium, provided you give appropriate credit to the original author(s) and the source, provide a link to the Creative Commons license, and indicate if changes were made. The Creative Commons Public Domain Dedication waiver ( http://creativecommons.org/publicdomain/zero/1.0/ ) applies to the data made available in this article, unless otherwise stated.
Reprints and permissions
About this article
Cite this article.
Kasetsirikul, S., Buranapong, J., Srituravanich, W. et al. The development of malaria diagnostic techniques: a review of the approaches with focus on dielectrophoretic and magnetophoretic methods. Malar J 15 , 358 (2016). https://doi.org/10.1186/s12936-016-1400-9
Download citation
Received : 19 April 2016
Accepted : 17 June 2016
Published : 12 July 2016
DOI : https://doi.org/10.1186/s12936-016-1400-9
Share this article
Anyone you share the following link with will be able to read this content:
Sorry, a shareable link is not currently available for this article.
Provided by the Springer Nature SharedIt content-sharing initiative
- Dielectrophoresis
- Magnetophoresis
- Cell deformability
- Cell morphology
Malaria Journal
ISSN: 1475-2875
- Submission enquiries: [email protected]

An official website of the United States government
The .gov means it's official. Federal government websites often end in .gov or .mil. Before sharing sensitive information, make sure you're on a federal government site.
The site is secure. The https:// ensures that you are connecting to the official website and that any information you provide is encrypted and transmitted securely.
- Publications
- Account settings
- Browse Titles
NCBI Bookshelf. A service of the National Library of Medicine, National Institutes of Health.
StatPearls [Internet]. Treasure Island (FL): StatPearls Publishing; 2024 Jan-.

StatPearls [Internet].
Emily Buck ; Nancy A. Finnigan .
Affiliations
Last Update: July 31, 2023 .
- Continuing Education Activity
Malaria is a parasitic infection transmitted by the Anopheles mosquito that leads to acute life-threatening disease and poses a significant global health threat. Two billion people risk contracting malaria annually, including those in 90 endemic countries and 125 million travelers. The Plasmodium parasite has a multistage lifecycle, which leads to characteristic cyclical fevers. With timely treatment, most people experience rapid resolution of symptoms; however, significant complications may occur, including cerebral malaria, severe malarial anemia, coma, or death. This activity reviews the epidemiology, presentation, and complications of Plasmodium malaria and the role of the interprofessional team in evaluating and managing patients with this life-threatening infection.
- Review the epidemiology of malaria infection.
- Describe the pathophysiology of malaria infection.
- Summarize the pharmacologic treatment strategies for malaria infection.
- Outline the importance of collaboration amongst an interprofessional team to improve outcomes for patients receiving malaria treatment.
- Introduction
Malaria is a parasitic infection transmitted by the Anopheles mosquito that leads to acute life-threatening disease and poses a significant global health threat. Two billion people risk contracting malaria annually, including those in 90 endemic countries and 125 million travelers, and 1.5 to 2.7 million people die in a year. [1] The Plasmodium parasite has a multistage lifecycle, which leads to characteristic cyclical fevers. With timely treatment, most people experience rapid resolution of symptoms; however, significant complications may occur, including cerebral malaria, severe malarial anemia, coma, or death. Preferred antimalarial therapeutic and chemoprophylactic regimens get dictated by species, geography, susceptibility, and patient demographics. Latent or reactivating infections may be reported years following exposure.
The incubation period, and therefore time to symptom development, varies by species: 8 to 11 days for P. falciparum , 8 to 17 days for P. vivax , 10 to 17 days for P. ovale , 18 to 40 days for P. malariae (though possibly up to several years), and 9 to 12 days for P. knowlesi . [1] The periodicity of the Plasmodium lifecycle creates the classic "malarial paroxysm" of rigors, followed by several hours of fever, followed by diaphoresis, and a drop to normal body temperature ( P. vivax infection establishes a 48-hour cycle), though this is less commonly seen today due to rapid identification and treatment. [1]
- Epidemiology
Forty percent of the global population resides in or visits malaria-endemic regions annually. [1] P. falciparum is present in Western and sub-Saharan Africa and displays the highest morbidity and mortality of the Plasmodia species. [2] P. vivax is present in South Asia, the Western Pacific, and Central America. [2] P. ovale and P. malariae are present in Sub-Saharan Africa. [2] P. knowlesi is present in Southeast Asia. [2] As many as 500 million malaria cases occur annually, with 1.5 to 2.7 million deaths. [1] Ninety percent of fatalities occur in Africa. [1] Those at highest risk include children under age 5, pregnant women, and disease naïve populations, including refugees in Central and Eastern Africa, nonimmune civilian and military travelers, and immigrants returning to their place of origin. [2]
Of the 125 million travelers who visit endemic locations each year, 10000 to 30000 develop malaria, and 1% of these will die from complications of their disease. [2] [3] Rising average global temperatures and changes in weather patterns are projected to expand the burden of malaria; a rise of 3 degrees Celsius is postulated to increase malaria incidence by 50 to 80 million. [1]
- Pathophysiology
Five Plasmodium species possess the ability to infect humans: P. falciparum, P. ovale, P. vivax, P. malariae , and P. knowlesi . [2] The female Anopheles mosquito ingests gametes during a blood meal, which form sporozoites that replicate in the gut. [1] During subsequent bloodmeals, saliva containing sporozoites gets released into a human host's bloodstream. [1] Within 60 minutes, sporozoites reach the liver, invade hepatocytes, and then rapidly divide, forming merozoites. In an active infection, organisms reenter the bloodstream and invade erythrocytes. [1] [4] Within erythrocytes, Plasmodia consume hemoglobin and develop from immature trophozoites (ring stage) to either mature trophozoites or gametocytes (CDC Malaria 2019). Mature trophozoites replicate, forming schizonts, disrupting erythrocyte cell membrane integrity, and leading to capillary endothelial adherence and cell lysis. [1]
Free heme is released into the peripheral blood, which stimulates endothelial activation. [5] [6] Untreated malaria lasts 2 to 24 months. [1] P. vivax and P. ovale infections may display "dormant schizogony," where inactive intrahepatic parasites (hypnozoites) remain until reactivation months to years in the future. [1] Although hypnozoite parasites do not routinely develop in the liver in the setting of P. falciparum and P. malariae infection, there are few reports of resurgent P. falciparum infection years after initial exposure. [7]
Pathogenesis stems from toxin-induced IFN-gamma and TNF-alpha secretion. [8] The innate immune response is dominated by monocyte and macrophage phagocytosis within the splenic red pulp. Adaptive immunity develops by IFN-gamma and TNF-alpha-induced class switching of CD4-positive lymphocytes. [4] TNF also suppresses hematopoiesis, which contributes to anemia. The liver and spleen enlarge, causing massive splenomegaly. [8]
Low arginine, low nitric oxide, and elevated arginase activity have been observed in severe malaria in peripheral blood. [9] Studies have shown that the parasite's arginase enzyme may contribute to low arginine in severely ill patients, thus reducing nitric oxide production. Low nitric may lead to subsequent pulmonary hypertension and myocardial wall stress in children. Therefore, peripheral arginine or inhaled nitric oxide are possible treatment options. [10]
Parasitemia dictates symptom onset and severity: symptoms typically develop with 0.002% parasitemia in naïve patients and 0.2% parasitemia in previously exposed patients. [1] Severe infection usually exhibits parasitemia of 5%. [1] [4]
- Histopathology
Intracellular digestion of hemoglobin by parasites forms hemozoin and makes the membrane less deformable, which results in hemolysis or splenic clearance.
- History and Physical
In taking a history, it is essential to inquire about the location of residence, recent travel and use of chemoprophylaxis, exposures (including sick contacts, fresh water, caves, farm/wild animals, insects/arthropods), HIV status, history of current or recent pregnancy, history of G6PD deficiency, history of sickle cell disease, history of anemia, history of blood or other cancers, and history of prior malarial infections (including successful or failed treatments).
Fever is the dominant symptom of malaria—fever, especially for seven or more days, in a patient residing in or with recent travel to an endemic region is highly suspicious and should prompt evaluation. [3] Adults may exhibit headaches, malaise, weakness, gastrointestinal distress, upper respiratory symptoms, and muscle aches; severe cases may include jaundice, confusion, seizures, and dark urine. [2] [1] Children are more likely to present with non-specific or gastrointestinal symptoms such as fever, lethargy, malaise, nausea, vomiting, abdominal cramps, and somnolence. [2] They are more likely to develop hepatomegaly, splenomegaly, and severe anemia without major organ dysfunction than adults. In the case of severe malaria, they present with more frequent seizures (60 to 80%), hypoglycemia, and concomitant sepsis but are less likely to develop pulmonary edema and renal failure than adults. [11] [2]
Pregnant Women
The clinical features of infection in pregnancy vary from asymptomatic to severe, depending on the degree of (incomplete) immunity that a woman had acquired by the time she got pregnant. In semi-immune pregnant women, only a few infections result in fever or other symptoms. [12] Malaria in pregnancy has a devastating effect on maternal health and has been associated with increased infant mortality due to low birth weight caused by either intrauterine growth restriction or preterm labor, or both. [12] P. falciparum infections are associated with complications such as maternal anemia, low birth weight, miscarriage, stillbirths, and congenital malaria. [13] [12] It is more likely for a pregnant woman in the second or third trimester to develop severe malaria with complications such as hypoglycemia and pulmonary edema compared to non-pregnant adults. [14]
Initial evaluation of undifferentiated fever in stable patients with possible malaria exposure includes a complete blood count, comprehensive metabolic panel, coagulation panel, blood culture, urinalysis, chest radiograph, and thick and thin blood smears. In patients with altered mental status, when cerebral malaria is suspected, a lactate level, arterial blood gas, and lumbar puncture may also be indicated. [2]
In patients with malaria, complete blood count reveals thrombocytopenia in 60-70% of all cases and varying degrees of anemia in 29% of adults and 78% of children. [2] Anemia is more severe in P. falciparum due to invasion of all aged erythrocytes and capillary and splenic erythrocyte sequestration secondary to decreased flexibility and cytoadherence. [1] Anemia is typically moderate with P. vivax and P. malariae due to preferential invasion of reticulocytes and older erythrocytes, respectively. [1] A comprehensive metabolic panel may reveal hepatocellular injury secondary to parasitic invasion, indirect hyperbilirubinemia due to hemolysis, electrolyte abnormalities secondary to the release of intracellular contents, concomitant dehydration, and kidney injury secondary to glomerular damage. [2] The coagulation panel may reveal coagulopathy concerning bleeding risk in patients with severe thrombocytopenia or liver dysfunction. Urinalysis may show proteinuria indicative of nephrotic syndrome. [1]
The gold standard for malaria diagnosis is a microscopic evaluation of Giemsa-stained thick and thin smears of a free-flowing venipuncture blood specimen. [2] [1] Examination with oil immersion must be completed at 100-times and 1000-times magnification to avoid missing low-level parasitemia or "delicate ring forms." [1] The extent of parasitemia is estimated by the number of organisms per high-powered field. [1] Varying microscopic appearance of infected erythrocytes guides speciation:
- The ring stage in P. falciparum appears as a "purple spot with a thin ring;" in P. vivax as a "purple spot with a deformed body;" in P. ovale as a "ring with a large purple spot;" in P. malariae as a "purple spot with a thick body;" and in P. knowlesi as a "purple spot (or spots) with an amorphous thick ring." [15]
- The trophozoite stage in P. falciparum appears as "a bigger spot [growing] around a smaller spot;" in P. vivax as "a misshapen circle which contains an extended spot;" in P. ovale as "an oval circle (sometimes with small corners) which contains a purple spot with undefined shapes;" in P. malariae as "basket or band-shaped [without a] spot;" and in P. knowlesi as a "purple branched spot." [15]
- The schizont stage in P. falciparum is not established; in P. vivax, it appears as "not defined purple spots inside a circle;" in P. ovale as "more than one spot inside an oval circle (sometimes with small corners);" in P. malariae as "diffuse purple spots around a darker spot;" and in P. knowlesi as "defined purple spots [that are] easy to count." [15]
- The gametocyte stage in P. falciparum appears as "banana [or] sausage-shaped;" in P. vivax as an "extended, big spot;" in P. ovale as a "row of accumulated spots;" in P. malariae as a "big stained spot which almost fills[s] the circle;" and in P. knowlesi as a "big spot which contains small spots." [15]
An initial negative smear does not rule out malaria, as infected erythrocytes may become intravascularly sequestered; if clinical suspicion of malaria is high, smears require repetition in 12 and 24 hours. [2] The malarial pigment in monocytes and neutrophils may also manifest on the blood smear, particularly in patients with cerebral malaria. [1]
Other diagnostic modalities include rapid diagnostic testing (RDT), microhematocrit centrifugation, and polymerase chain reaction (PCR). RDTs detecting parasitic antigens histidine-rich-protein-2, lactate dehydrogenase, and aldolase are increasingly being utilized to diagnose P. falciparum infection. [2] [16] Sensitivities approach 100%, though microscopy is still a recommendation at the time of presentation and 12 and 24 hours. Limitations of RDTs include the detection of P. falciparum species only, the inability to quantify parasitic burden, and false-positive results occurring weeks after infection due to persistent blood antigens. [2] Microhematocrit centrifugation isolates infected erythrocytes, then binds to acridine in the collection tube, causing the fluorescence of parasites. [1] PCR is useful in low-level parasitemia detection and speciation.
- Treatment / Management
Treatment for patients diagnosed with malaria includes schizonticidal medications, supportive care, and hospitalization for high-risk patients. Naïve adult and pediatric patients receiving active antimalarial treatment should remain inpatient for at least 24 hours to ensure adequate and correctly timed medication dosing and to trend parasitemia to evaluate treatment response. Higher initial parasitemia and poor downtrend are associated with fluid imbalance, renal dysfunction, and respiratory distress syndrome. [2] Unstable patients, particularly those with cerebral malaria or significant respiratory sequelae, require intensive care. [2]
Treatment involves combination therapy targeting both the hepatic and erythrocytic forms. [17] The chief antimalarials are chloroquine, hydroxychloroquine, primaquine, artemisinin-based combination therapy (ACT), and atovaquone-proguanil. Chloroquine and hydroxychloroquine are synthetic forms of quinine. [18] [19] They disrupt the erythrocytic stage by interfering with parasitic hemoglobin metabolism and increasing intracellular pH. [18] [19] They generally require two days of treatment, allowing for better tolerance and shorter admissions. [2] However, chloroquine may enhance gametogenesis, contributing to resistance, which is a concern, particularly in South Asia. [17] Primaquine is a hypnozointocidal agent added for P. vivax or P. ovale infection for the eradication of liver parasites and the prevention of dormancy and relapse. [2] [20]
Primaquine is contraindicated in pregnant and G6PD deficient patients due to fetal teratogenicity and hemolytic reaction (will see bite cells and Heinz bodies on blood smear), respectively. [3] Artemisinins are active against all parasite lifecycle stages. [2] Atovaquone targets the cellular electron transport chain inhibiting ATP production; proguanil enhances atovaquone’s effect by sensitizing parasitic mitochondria. [21] Atovaquone-proguanil is active against the erythrocytic and extraerythrocytic forms. [17] [21]
Per the 2019 CDC Guidelines below, appropriate treatment depends on the Plasmodium species, clinical stability, age of the patient, and regional antimalarial susceptibility:
- Uncomplicated P. falciparum, P. malariae or P. knowlesi infections in chloroquine-sensitive regions are treated with a chloroquine phosphate 600 mg (pediatric: 10 mg/kg) loading dose, followed by 300 mg (pediatric: 5 mg/kg) at 6, 24, 48 hours; or a hydroxychloroquine 620 mg (pediatric: 10 mg/kg) loading dose, followed by 310 mg (pediatric: 5 mg/kg) at 6, 24, and 48 hours.
- Uncomplicated P. falciparum infections in chloroquine-resistant or unknown regions are treated with atovaquone-proguanil 250 mg/100 mg 4 tabs (pediatric: varied weight-based dosing, 6.5 mg/25 mg tabs) daily for 4 days; or artemether-lumefantrine 20 mg/120 mg 4 tabs (pediatric: varied weight-based tabs) at initial dose, then 8 hours later, then twice daily for 2 days; or quinine sulfate 542 mg (pediatric: 8.3 mg/kg) three times daily for 3 days (7 days if in Southeast Asia) plus either doxycycline 100 mg daily for 7 days (pediatrics 2.2 mg/kg every 12 hours), or tetracycline 250 mg daily for 7 days (pediatric: 25 mg/kg/day divided four times daily for 7 days), or clindamycin 20 mg/kg/day divided three times daily for 7 days (pediatric: same); or mefloquine 684 mg (pediatric: 13.7 mg/kg) loading dose followed by 456 mg (pediatric: 9.1 mg/kg) every 6 to 12 hours for total of 1250 mg (pediatric total: 25 mg/kg).
- Uncomplicated P. vivax or P. ovale infections in chloroquine-sensitive regions receive treatment with chloroquine phosphate or hydroxychloroquine as per above, plus either primaquine phosphate 30 mg (pediatric: 0.5 mg/kg) daily for 14 days or tafenoquine 300 mg once (same in children older than 16 years).
- Uncomplicated P. vivax infections in chloroquine-resistant regions (Indonesia, Papua New Guinea) get treated with quinine sulfate as per above plus either doxycycline, primaquine, or tafenoquine as per above; or atovaquone-proguanil as per above plus either primaquine or tafenoquine; or mefloquine as per above plus either primaquine or tafenoquine as per above.
- Uncomplicated infections with any species in pregnant women in chloroquine-sensitive regions require treatment with chloroquine or hydroxychloroquine as per above.
- Uncomplicated infections with any species in pregnant women in chloroquine-resistant regions are treated with quinine sulfate as per above plus either clindamycin or mefloquine as per above in the first, second, or third trimesters; or artemether-lumefantrine as per above in only the second and third trimesters.
- Severe malaria infection in unstable, non-pregnant patients in all regions includes IV artesunate 2.4 mg/kg (pediatric: children greater than 20 kg receive 2.4 mg/kg, children less than 20 kg receive 3.0 mg/kg) at 0, 12, 24, and 48 hours and either artemether-lumefantrine, atovaquone-proguanil, doxycycline, or mefloquine as per above.
- Differential Diagnosis
The differential for undifferentiated fever is extremely broad and varies based on geographic location and age. In a 2017 review of fever in returning travelers, 77% had protozoal malaria, 18% had a bacterial enteric fever ( Salmonella enterica, typhi, or paratyphi ), and 5% had another infection. In patients presenting with fever and significant somnolence or seizures, viral or bacterial meningitis or meningoencephalitis must remain on the differential and prompt consideration of lumbar puncture. [2] [22] Viral etiologies include avian influenza, Middle East respiratory syndrome coronavirus, hemorrhagic fever (Ebola virus, Lassa fever, Marburg hemorrhagic fever, Crimean-Congo hemorrhagic fever), yellow fever, dengue, Japanese encephalitis, Rift Valley fever, hepatitis virus (A or B), viral gastroenteritis, and rabies. [22] Bacterial etiologies include anthrax, epidemic typhus, ehrlichiosis, leptospirosis, melioidosis, murine (endemic) typhus, spotted fever group rickettsioses, Q fever, and Yersinia pestis. [22] [2]
The differential in children varies by region, with the most likely etiology being a viral or bacterial infection. In a 2014 study of febrile children in a tropical region, 10.5% were diagnosed with malaria, 62% were diagnosed with a respiratory infection, 13.3% with a systemic bacterial infection (usually staphylococcus or streptococcus bacteremia), and 10.3% with gastroenteritis (viral or bacterial). [23] Urinary tract infection and typhoid may also be considerations. Meningitis must be ruled out in somnolent children. [23]
- Treatment Planning
Artemether 20 mg/ lumefantrine 120 mg Artemether 40 mg/ lumefantrine 240 mg
Table 1. Artemisinin combination therapy (ACT) regimens for treatment of uncomplicated Plasmodium falciparum malaria in nonpregnant adults and children
Quinine: 542 mg base (= 650 mg salt) three times daily for a weekClindamycin: 20 mg base/kg/day (up to 1.8 grams) divided three times daily for a week OR Artemisinin combination therapy can be used as an alternate therapy in the first trimester if the above (more...)
Table 2. Oral regimens for treatment of Plasmodium falciparum malaria in pregnant women regions with c hloroquine-resistant P. falciparum infection.
00 hours: 600 mg base (= 1000 mg salt) 06 hours: 300 mg base (= 500 mg salt)
Table 3. Oral regimens for treatment of Plasmodium falciparum malaria in pregnant women in regions with c hloroquine-sensitive P. falciparum infection
The duration of untreated infection and time to relapse vary by location and species. P. falciparum and P. ovale infections last 2 to 3 weeks and may relapse 6 to 18 months later, usually from a new primary infection. [1] P. vivax infection lasts 3 to 8 weeks and may relapse months to up to 5 years later. [1] P. malariae infection lasts 3 to 24 weeks and may relapse up to 20 years later. [1]
Relapse is a case of recurrent symptoms months to years after the resolution of erythrocytic organisms due to reinfection or hypnozoite activation. [2] [1] Recrudescence is defined as recurrent symptoms within days to weeks of acute illness due to remaining parasitemia after ineffective or incomplete treatment or failed host immune response, more commonly in P. falciparum . [2] [1] Appropriate, complete treatment usually results in a full resolution of symptoms.
The two main determinants reflecting the outcome for both adults and children were the level of consciousness assessed by coma scales and the degree of metabolic acidosis, assessed clinically by breathing pattern or, more precisely, with measurement of bicarbonate, base deficit, and plasma lactate. [32] While the general mortality of treated severe malaria is between 10 to 20%, the mortality in pregnant women reaches approximately 50%. [14]
- Complications
The significant complications of malaria are cerebral malaria, severe malarial anemia, and nephrotic syndrome (NS).
Cerebral malaria accounts for 80% of fatal malaria cases, most often occurring with P. falciparum infection. [1] It presents as slow-onset altered mental status, violent behavior, headache, and extremely high fever (up to 42 degrees C), followed by coma, metabolic acidosis, hypoglycemia, and possibly seizures and death. [1] [4] It most commonly affects children under age 5, with a case fatality rate of 18%. [33] Pathogenesis involves malarial rosettes (one infected erythrocyte surrounded by three uninfected erythrocytes), causing cerebral sequestration and vasodilation, as well as excessive oxygen free radicals, IFN-gamma, and TNF-alpha leading to an extreme inflammatory response. [1] [4] [33] This leads to congestion, decreased perfusion, endothelial activation, impairment of the blood-brain barrier, and cerebral edema, which increases brain volume. [33]
Increased brain volume is the major contributor to mortality in cerebral malaria. In a 2015 study of Malawian children with cerebral malaria, 84% of those who died had severely increased brain volume on MRI; children who survived showed lower initial brain volume or a downtrend over time. [33]
Severe malarial anemia stems from TNF-alpha-mediated mechanisms involving both increased destruction and decreased production of erythrocytes, including cell lysis as parasites replicate and exit erythrocytes, splenic removal and autoimmune lysis of immune-marked erythrocytes, poor iron incorporation into new heme molecules, and bone marrow suppression during severe infection leading to decreased production. [1] [4] Blackwater fever is severe anemia with hemoglobinuria and renal failure in the context of "massive intravascular hemolysis" in the setting of repeat P. falciparum infections treated with chronic quinine; it is rare and thought to be associated with G6PD deficiency. [34]
Nephrotic syndrome occurs secondary to glomerular antigen-antibody complex deposition and presents similarly to membranoproliferative glomerulonephritis with proteinuria and decreased renal function, which may lead to renal failure. Nephrotic syndrome is common in P. malariae and P. knowlesi , possible in P. vivax , and rare in P. falciparum and P. ovale infections. [1]
Additional complications include:
- Bilious remittent fever presents with abdominal pain and persistent vomiting that may lead to severe dehydration, jaundice, and dark urine.
- Algid malaria is an adrenal insufficiency due to parasitic congestion and subsequent necrosis of the adrenal glands.
- Acute respiratory distress syndrome, circulatory collapse, disseminated intravascular coagulation, pulmonary edema, coma, and death. [1]
Malaria infection during pregnancy may result in low birth weight or fetal demise. [1]
- Consultations
Recommended consultations for non-infectious disease experts in the management or prevention of malaria include infectious disease and preventive or travel medicine.
- Deterrence and Patient Education
The recommendation is that patients schedule a pre-travel appointment with a preventive medicine or infectious disease physician for education regarding malaria deterrence. Malaria prevention centers around vector control and chemoprophylaxis while exposed to mosquito-ridden environments.
Vector control is the prevention of mosquito bites by way of insecticide-impregnated bed nets, permethrin treatment of clothing, and DEET application to the skin. [3] The three main prophylactic agents for Plasmodium falciparum are atovaquone-proguanil, doxycycline, and mefloquine. Atovaquone-proguanil is taken once daily during and one week after travel to an endemic region; it suppresses the hepatic stage and does not have approval for pregnancy. [2] Doxycycline is taken once daily during and one month after travel; it suppresses the blood stage. [2] Doxycycline has the added benefit of prophylaxis against Rickettsial disease, Q fever, leptospirosis, and travelers’ diarrhea; however, it may cause gastrointestinal distress, photosensitivity, and increased risk of candida infection. Mefloquine is taken once weekly during and one month after travel; it suppresses the blood stage. [2] It has the benefit of safety in the second and third trimester of pregnancy; however, it has a far higher risk of neuropsychiatric side effects. [2] The US military primarily utilizes doxycycline if susceptibilities are equal. [2] For pregnant women in the first trimester or breastfeeding women, chloroquine or mefloquine prophylaxis are preferable; data regarding the safety of atovaquone-proguanil prophylaxis in pregnancy is limited. [35]
- Enhancing Healthcare Team Outcomes
The timely care of patients diagnosed with malaria and clinically relevant research regarding advancing diagnostic techniques and treatment requires interprofessional teamwork and communication between clinicians, infectious disease experts, pharmacists, nurses, and global health professionals.
Any clinician treating malaria will initiate treatment as outlined above. Still, it is good policy to include an infectious disease specialist and involve an infectious disease board-certified pharmacist, who can also examine the regimen and agents chosen, as well as verify dosing and drug interactions. A nurse with infectious disease specialty training can also help by answering patient questions, serving as a bridge to the treating clinician, and monitoring treatment progress and potential adverse drug reactions. All team members must keep accurate and updated records, so everyone involved in treatment has the same information on the patient's case. If there are any concerns, each team member must be free to communicate with other team members so that appropriate interventions can be started or therapeutic modifications can be implemented. This collaborative interprofessional approach can optimize outcomes for malaria patients. [Level 5]
- Review Questions
- Access free multiple choice questions on this topic.
- Comment on this article.
Life Cycle of the Malaria Parasite Contributed by Wikimedia Commons, National Institutes of Health (NIH) (Public Domain)
Aedes species mosquito Image courtesy of S Bhimji MD
Blood smear malaria Image courtesy S Bhimji MD
Table 1 - Diagnostic criteria for severe P.falciparum malaria. Contributed by Lara Zekar, MD
Disclosure: Emily Buck declares no relevant financial relationships with ineligible companies.
Disclosure: Nancy Finnigan declares no relevant financial relationships with ineligible companies.
This book is distributed under the terms of the Creative Commons Attribution-NonCommercial-NoDerivatives 4.0 International (CC BY-NC-ND 4.0) ( http://creativecommons.org/licenses/by-nc-nd/4.0/ ), which permits others to distribute the work, provided that the article is not altered or used commercially. You are not required to obtain permission to distribute this article, provided that you credit the author and journal.
- Cite this Page Buck E, Finnigan NA. Malaria. [Updated 2023 Jul 31]. In: StatPearls [Internet]. Treasure Island (FL): StatPearls Publishing; 2024 Jan-.
In this Page
Bulk download.
- Bulk download StatPearls data from FTP
Related information
- PMC PubMed Central citations
- PubMed Links to PubMed
Similar articles in PubMed
- Folic acid supplementation and malaria susceptibility and severity among people taking antifolate antimalarial drugs in endemic areas. [Cochrane Database Syst Rev. 2022] Folic acid supplementation and malaria susceptibility and severity among people taking antifolate antimalarial drugs in endemic areas. Crider K, Williams J, Qi YP, Gutman J, Yeung L, Mai C, Finkelstain J, Mehta S, Pons-Duran C, Menéndez C, et al. Cochrane Database Syst Rev. 2022 Feb 1; 2(2022). Epub 2022 Feb 1.
- Malaria Surveillance - United States, 2017. [MMWR Surveill Summ. 2021] Malaria Surveillance - United States, 2017. Mace KE, Lucchi NW, Tan KR. MMWR Surveill Summ. 2021 Mar 19; 70(2):1-35. Epub 2021 Mar 19.
- Malaria Surveillance - United States, 2016. [MMWR Surveill Summ. 2019] Malaria Surveillance - United States, 2016. Mace KE, Arguin PM, Lucchi NW, Tan KR. MMWR Surveill Summ. 2019 May 17; 68(5):1-35. Epub 2019 May 17.
- Review [The reemergence of malaria in Israel?]. [Harefuah. 2004] Review [The reemergence of malaria in Israel?]. Anis E, Pener H, Goldmann D, Leventhal A. Harefuah. 2004 Nov; 143(11):815-9, 838, 837.
- Review Diagnosis and Treatment of the Febrile Child. [Reproductive, Maternal, Newbor...] Review Diagnosis and Treatment of the Febrile Child. Herlihy JM, D’Acremont V, Hay Burgess DC, Hamer DH. Reproductive, Maternal, Newborn, and Child Health: Disease Control Priorities, Third Edition (Volume 2). 2016 Apr 5
Recent Activity
- Malaria - StatPearls Malaria - StatPearls
Your browsing activity is empty.
Activity recording is turned off.
Turn recording back on
Connect with NLM
National Library of Medicine 8600 Rockville Pike Bethesda, MD 20894
Web Policies FOIA HHS Vulnerability Disclosure
Help Accessibility Careers
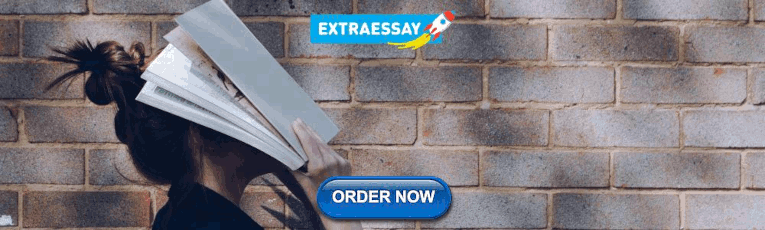
IMAGES
VIDEO
COMMENTS
Malaria is a mosquito-borne infectious disease of humans and other animals caused by. protists (a type of microorganism) of the genus Plasmodium. It begins with a bite from an. infected female ...
PDF | Malaria is a global public health burden with an estimated 229 million cases reported worldwide in 2019. ... Discover the world's research. 25+ million members ... This paper was uploaded to ...
1. Introduction. Malaria affected an estimated 219 million people causing 435,000 deaths in 2017 globally. This burden of morbidity and mortality is a result of more than a century of global effort and research aimed at improving the prevention, diagnosis, and treatment of malaria [].Malaria is the most common disease in Africa and some countries in Asia with the highest number of indigenous ...
View PDF A case of severe Plasmodium ovale malaria with acute respiratory distress syndrome and splenic infarction in a male traveller presenting in Italy. Plasmodium ovale malaria is usually considered a tropical infectious disease associated with low morbidity and mortality. However, severe disease and death have previously been reported.
Further research on the use of mAbs against malaria is needed and should be performed in endemic areas and include at risk groups such as under 5s and pregnant women. The future role of mAbs for malaria prevention will depend on accessibility and the ultimate overall cost of implementation. ... •Malaria vaccine: WHO position paper - March ...
5.6eported malaria cases in R HBHI countries since 2018 and comparisons with estimated cases 48 6.vestments in malaria programmes and research In 52 6.1unding trends for malaria control and elimination 52F 6.2vestments in malaria-related R&D 56In 7.istribution and coverage of malaria prevention, diagnosis and treatment D 58
The World malaria report 2022 provides comprehensive data and analysis on the global, regional and country-level progress and challenges in the fight against malaria. It covers topics such as antimalarial drug resistance, urban malaria, malaria vaccine and humanitarian emergencies. Download the full report in PDF format from iris.who.int.
This paper aims to be a didactic material providing the reader with an overview of malaria. More importantly, using a system approach lens, we intend to highlight the debated topics and the multifaceted thematic aspects of malaria transmission mechanisms, while showing the control approaches used as well as the model supporting the dynamics of ...
6. Investments in malaria programmes and research 44 6.1 Funding trends for malaria control and elimination 45 6.2 Global trends in real GDP growth and out-of-pocket health expenditure 54 6.3 Investments in malaria-related R&D 58 7. Distribution and coverage of malaria prevention, diagnosis and treatment 60 7.1 Distribution and coverage of ITNs 60
Malaria is a life-threatening febrile illness caused by Plasmodium spp. parasites that are transmitted to humans by infected female Anopheles mosquitoes. According to WHO, 241 million cases ...
Great progress has been made in recent years to reduce the high level of suffering caused by malaria worldwide. Notably, the use of insecticide-treated mosquito nets for malaria prevention and the use of artemisinin-based combination therapy (ACT) for malaria treatment have made a significant impact. Nevertheless, the development of resistance to the past and present anti-malarial drugs ...
For further reading, the following articles, referenced in this video, are available at the Journal's website: A Malaria Vaccine for Africa — An Important Step in a Century-Long Quest, in the ...
Methods: This review considers data from peer‑reviewed articles and information from the National Malaria Control Programme reports, based on field investigations or samples collected from 1992 to 2015. Peer‑reviewed papers were searched throughout online bibliographic databases PubMed, HINARI and Google Scholar using the following terms:
Download PDF. OUTLOOK; 28 June 2023; Malaria: highlights from research ... To test this hibernation hypothesis, researchers led by Alpha Yaro at the Malaria Research and Training Center in Bamako ...
Malaria is resurging in many African and South American countries, exacerbated by COVID-19-related health service disruption. In 2021, there were an estimated 247 million malaria cases and 619 000 deaths in 84 endemic countries. Plasmodium falciparum strains partly resistant to artemisinins are entrenched in the Greater Mekong region and have emerged in Africa, while Anopheles mosquito vectors ...
Severe malaria is a medical emergency. It is a major cause of preventable childhood death in tropical countries. Severe malaria justifies considerable global investment in malaria control and elimination yet, increasingly, international agencies, funders and policy makers are unfamiliar with it, and so it is overlooked. In sub-Saharan Africa, severe malaria is overdiagnosed in clinical practice.
GOALS, MILESTONES AND TARGETS FOR THE GLOBAL TECHNICAL STRATEGY FOR MALARIA 2016-2030. VISION - A WORLD FREE OF MALARIA GOALS MILESTONES TARGETS. 2020 2025 2030 1 . Reduce malaria mortality rates globally compared with 2015 At least 40% At least 75% At least 90% 2 .
Malaria is a mosquito-borne disease that is caused by Plasmodium parasites. Patients with malaria experience flu-like symptoms and, in severe cases, the disease can progress to neurological ...
ward" was made in malaria research, particularly in the fields ofimmuneresponse and chemotherapy inasmuch as a great manycompounds were tested. Ananalysis oftheliterature indicated that, during the period 1971-73, at least 800 papers on malaria research were published. The total number of such papers is nodoubtconsiderably greater, as acertain
CDC's strategic research helped develop and evaluate each of the effective tools now used throughout the world to prevent and control malaria: Massive scale-up of these proven interventions in the last decade has led to unprecedented gains in the fight against malaria. From 2000 to 2012, 3.3 million lives were saved globally, and malaria ...
The large number of deaths caused by malaria each year has increased interest in the development of effective malaria diagnoses. At the early-stage of infection, patients show non-specific symptoms or are asymptomatic, which makes it difficult for clinical diagnosis, especially in non-endemic areas. Alternative diagnostic methods that are timely and effective are required to identify ...
Methods: In this paper, the international scientific publication on malaria and P. vivax has been analyzed using the Scopus database to try to define global trends in this field of study. Results: It has been shown that events such as the emergence of resistance to certain drugs can break a trend.
malaria, which can result in stillbirths or spontaneous abortion or in the death of the mother (Luxemburger et al., 1997). In areas of high trans- ... Research to assess the safety, effi cacy and programme feasibility of other antimalarials in intermittent preventive treatment is under way. 3
Malaria is a parasitic infection transmitted by the Anopheles mosquito that leads to acute life-threatening disease and poses a significant global health threat. Two billion people risk contracting malaria annually, including those in 90 endemic countries and 125 million travelers, and 1.5 to 2.7 million people die in a year.[1] The Plasmodium parasite has a multistage lifecycle, which leads ...