Thank you for visiting nature.com. You are using a browser version with limited support for CSS. To obtain the best experience, we recommend you use a more up to date browser (or turn off compatibility mode in Internet Explorer). In the meantime, to ensure continued support, we are displaying the site without styles and JavaScript.
- View all journals
- Explore content
- About the journal
- Publish with us
- Sign up for alerts
Focus 14 March 2024
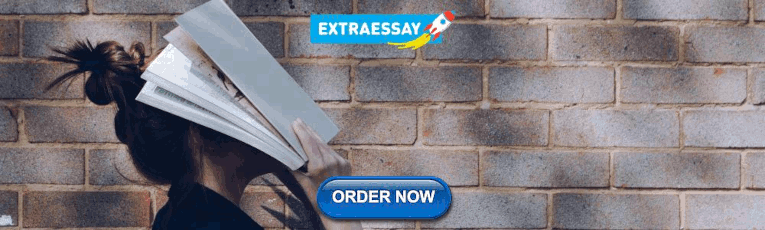
Physics Education Research
Using evidence-based approaches to improve the teaching of physics can help students achieve more and improve equity. In this Focus Issue, we survey the current state of this research field.
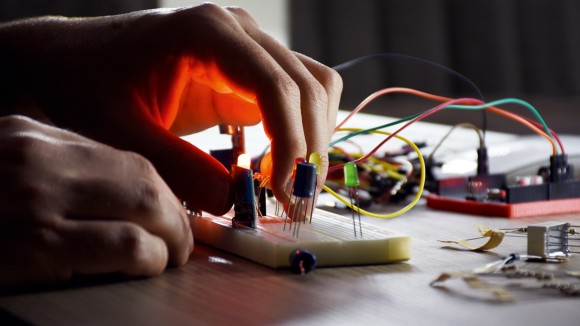
Elizaveta Dubrovina
Senior Editor, Nature Physics
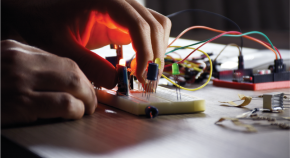
Unlock the potential of a physics education
This month in Nature Physics , we publish a Focus issue that highlights the importance of physics education research.
Reviews and Perspectives
Towards meaningful diversity, equity and inclusion in physics learning environments.
Women and ethnic and racial minority students are underrepresented in physics. This Review summarizes research on equity and inclusion in physics education and makes recommendations for making physics learning environments more equitable.
- Alexandru Maries
- Chandralekha Singh
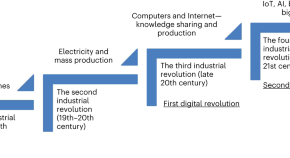
Epistemic agency as a critical mediator of physics learning
Encouraging students to take ownership of their learning can improve their outcomes. This Perspective discusses ways to achieve this in the context of physics education and how digital technology can help Gen Z students in particular.
- Nam-Hwa Kang
Racial equity in physics education research
Injustices and oppression are pervasive in society, including education. An intersectional, equity-oriented approach can help remove systemic obstacles and improve the experience of marginalized people in physics education through decolonial and critical race lenses.
- Geraldine L. Cochran
- Simone Hyater-Adams
- Ramón S. Barthelemy
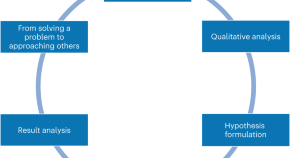
A physics curriculum for the modern world
Increasingly, physics graduates take jobs outside academia. Active teaching approaches lead to deeper conceptual understanding and a more varied skill set and are therefore more likely to prepare students for successful careers.
- Jenaro Guisasola
- Kristina Zuza
Computing in physics education
Computing is central to the enterprise of physics but few undergraduate physics courses include it in their curricula. Here we discuss why and how to integrate computing into physics education.
- Marcos D. Caballero
- Tor Ole B. Odden
Quick links
- Explore articles by subject
- Guide to authors
- Editorial policies


Physics Education Research
Physics Education Research (PER) is the study of how people learn physics and how to improve the quality of physics education. Researchers use the tools and methods of science to answer questions about physics learning that require knowledge of physics. Researchers focus on developing objective means of measuring the outcomes of educational interventions. How do we know whether our courses and interventions are successful?
One such approach is the design of diagnostic assessments and surveys. While many instructors develop questions to assess student learning, diagnostic research assessments undergo rigorous design, testing, and validation processes to facilitate objective comparisons between students and methods of instruction. These assessments are like detectors that must be carefully crafted and calibrated to ensure we understand what they are measuring.
The Cornell Physics Education Research Lab has a large focus on studying and developing learning in lab courses. Researchers are collecting data to evaluate the efficacy of lab courses in achieving various goals, from reinforcing physics concepts to fostering student attitudes and motivation to developing critical thinking and experimentation skills. They are designing innovative teaching methods to harness the affordances of lab courses, namely, working with messy data, getting hands on materials, troubleshooting equipment, and connecting physical models to the real world and data. There are many open research questions related to understanding how students learn these ideas.
This work will be facilitated by a research Active Learning Initiative grant from the Cornell University College of Arts and Sciences led by Natasha Holmes (PI). This grant will facilitate the renewal of the physics lab elements of the two calculus-based introductory physics course sequences. In addition to redesigning the instructional materials, this project will involve significant attention on understanding how instructional materials get passed down between instructors and sustained over time, how teaching assistants are trained to support the innovative designs, and many open research questions to evaluate students’ experience and learning in these courses.
The recent Cornell University Physics Initiative in Deliberate practice (CUPID) was a 5-year project to renew the introductory, calculus-based physics course sequence for Engineering and Physics majors. This project, led by Jeevak Parpia and Tomás Arias and involving more than 8 other faculty and lecturers in the department, applied results of PER to improve the teaching and learning in Cornell University courses, and to test the generalizability of results observed elsewhere. By collecting assessment, survey, and exam data across the duration of the course implementation, the group demonstrated significant improvements in student learning and attitudes. They are now in the process of monitoring how the course materials get passed on to new faculty. There are many opportunities to study differences in various forms of active learning.
Related people
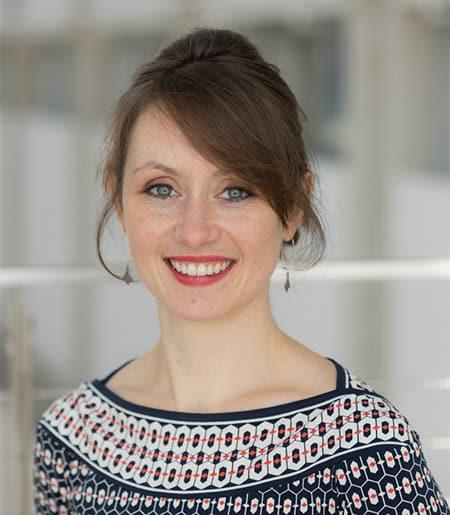
Ann S. Bowers Associate Professor
All research areas
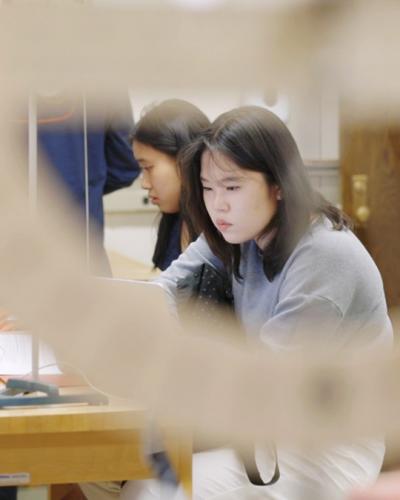
Advertisement
Teaching–Learning Contemporary Physics: from Research to Practice
- Published: 07 October 2022
- Volume 22 , pages 1002–1005, ( 2022 )
Cite this article
- Sri Wahyuni ORCID: orcid.org/0000-0002-0454-0640 1 ,
- Andreas Suparman ORCID: orcid.org/0000-0001-8922-8340 1 &
- Windy Kasmita ORCID: orcid.org/0000-0002-6297-827X 1
210 Accesses
1 Altmetric
Explore all metrics
As part of Springer, the edited book addresses innovative approaches and pedagogical strategies, the development of effective methods for assessing, and the innovative project in teaching and learning contemporary physics. This volume includes successful experimental and theoretical studies of QM teaching, which the experts or teachers can then adopt or modify the concept into practice in context. The 19 chapters, representing results of original study works dealing with Physics Education, are selected from the contributions in a conference on the teaching and learning of quantum physics. The goal of the book is to introduce contemporary physics to all level schools. Without a doubt, the book is recommended for high school teachers, instructional developers, and scientists to explore and develop the theories, approaches, and strategies in the book to advance teaching/learning of CP.
Cet ouvrage révisé publié chez Springer traite des approches et des stratégies pédagogiques innovantes, du développement de méthodes d’évaluation efficaces et du projet novateur dans l’enseignement et l’apprentissage de la physique contemporaine (PC). Ce volume comprend des études expérimentales et théoriques concluantes sur l’enseignement de la PC, que les experts ou les enseignants peuvent ensuite adopter ou modifier dans la mise en pratique contextuelle du concept. Les 19 chapitres, qui représentent les résultats d’études originales traitant de l’enseignement de la physique, sont sélectionnés parmi les contributions faites lors d’une conférence sur l’enseignement et l’apprentissage de la physique quantique. L’objectif du livre est d’introduire la physique contemporaine dans les écoles, et ce à tous les niveaux. Il est sans aucun doute recommandé aux enseignants du secondaire, aux développeurs de systèmes éducatifs et aux scientifiques d’explorer et d’approfondir les théories, les approches et les stratégies présentées dans l’ouvrage afin de faire progresser l’enseignement et l’apprentissage de la PC.
This is a preview of subscription content, log in via an institution to check access.
Access this article
Price includes VAT (Russian Federation)
Instant access to the full article PDF.
Rent this article via DeepDyve
Institutional subscriptions
Similar content being viewed by others
Social Learning Theory—Albert Bandura
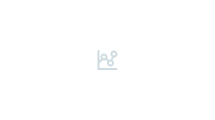
Evolution and Revolution in Artificial Intelligence in Education
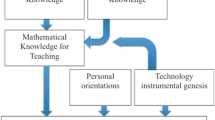
Teaching with digital technology
Jarosievitz, B., & Sükösd, C. (2021). Teaching-Learning Contemporary Physics: From Research to Practice. Springer: Switzerland.
Book Google Scholar
Stern, C., Echeverria, C., & Porta, D. (2017). Teaching Physics Through Experimental Project . Procedia IUTAM. https://doi.org/10.1016/j.piutam.2017.03.026 .
Article Google Scholar
Download references
Acknowledgements
The authors would like to express their deepest gratitude to Lembaga Pengelolaan Dana Pendidikan (LPDP/Indonesia Endowment Fund for Education) under the Ministry of Finance of the Republic of Indonesia as the sponsor for their masters’ studies, and the support of this publication. We thank our mentors Fikri Yanda, Frida Akmalia, and Yunita Laila Zulfa from Universitas Pendidikan Indonesia for mentoring and editing the manuscript.
Author information
Authors and affiliations.
Universitas Pendidikan Indonesia, Jl. Dr. Setiabudi No. 229, Kelurahan Isola, Kecamatan Sukasari, Kota Bandung, 4015, Indonesia
Sri Wahyuni, Andreas Suparman & Windy Kasmita
You can also search for this author in PubMed Google Scholar
Corresponding author
Correspondence to Sri Wahyuni .
Ethics declarations
Conflict of interest.
The authors declare no competing interests.
Additional information
Publisher's note.
Springer Nature remains neutral with regard to jurisdictional claims in published maps and institutional affiliations.
Rights and permissions
Springer Nature or its licensor holds exclusive rights to this article under a publishing agreement with the author(s) or other rightsholder(s); author self-archiving of the accepted manuscript version of this article is solely governed by the terms of such publishing agreement and applicable law.
Reprints and permissions
About this article
Wahyuni, S., Suparman, A. & Kasmita, W. Teaching–Learning Contemporary Physics: from Research to Practice. Can. J. Sci. Math. Techn. Educ. 22 , 1002–1005 (2022). https://doi.org/10.1007/s42330-022-00233-2
Download citation
Accepted : 17 September 2022
Published : 07 October 2022
Issue Date : December 2022
DOI : https://doi.org/10.1007/s42330-022-00233-2
Share this article
Anyone you share the following link with will be able to read this content:
Sorry, a shareable link is not currently available for this article.
Provided by the Springer Nature SharedIt content-sharing initiative
- Quantum mechanics (QM)
- Contemporary physics (CP)
- Modern Physics
- Innovative approach
- Active learning
- All level education
- Innovation in learning
- Find a journal
- Publish with us
- Track your research
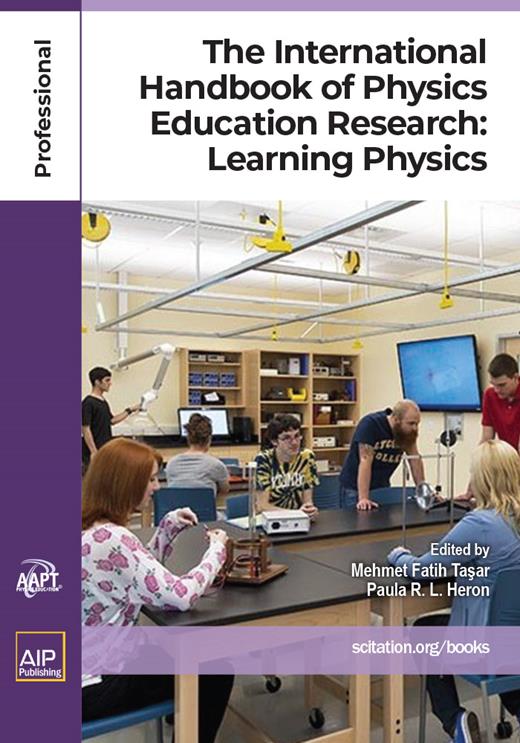
The International Handbook of Physics Education Research: Learning Physics
Mehmet Fatih Taşar, Ph.D. , of Georgia State University is a physics education researcher who has contributed to the field in various lead capacities. He taught physics and conducted research at many prestigious institutions including Penn State University, RMIT University, Gazi University, and Georgia State University. He has supervised graduate students to successful completion of their degrees and organized many international conferences such as WCPE, ESERA , and iSER . Dr. Taşar has served as an editor for scholarly journals including EJMSTE, IJPCE, Hellenic Journal of STEM , and ARISE .
Paula R. L. Heron, Ph.D. , is Professor of Physics at the University of Washington where she has been teaching since 1995. She is co-founder and co-chair of the biannual “Foundations and Frontiers in Physics Education Research” conference series, and she has been a committee executive for groups within the American Physical Society (APS), American Association of Physics Teachers (AAPT), and National Research Council. She serves as an associate editor of Physical Review (APS). She was elected a Fellow of APS in 2007 and 2008.
- Book Chapters
- Figures & tables
- Supplementary Data
- Peer Review
- Search Site
- Cite Icon Cite
The International Handbook of Physics Education Research: Learning Physics Edited by: Mehmet Fatih Taşar, Paula R. L. Heron https://doi.org/10.1063/9780735425477 ISBN (print): 978-0-7354-2544-6 ISBN (electronic): 978-0-7354-2547-7 Publisher: AIP Publishing LLC
Download citation file:
- Ris (Zotero)
- Reference Manager
Table of Contents
- Front Matter By Mehmet Fatih Taşar ; Mehmet Fatih Taşar Georgia State University , Atlanta, GA, USA Search for other works by this author on: This Site PubMed Google Scholar Paula R. L. Heron Paula R. L. Heron University of Washington , Seattle, WA, USA Search for other works by this author on: This Site PubMed Google Scholar Doi: https://doi.org/10.1063/9780735425477_frontmatter Abstract View Chapter Open the PDF Link PDF for Front Matter in another window
- Introduction By Mehmet Fatih Taşar ; Mehmet Fatih Taşar Georgia State University , Atlanta, GA, USA Search for other works by this author on: This Site PubMed Google Scholar Paula R. L. Heron Paula R. L. Heron University of Washington , Seattle, WA, USA Search for other works by this author on: This Site PubMed Google Scholar Doi: https://doi.org/10.1063/9780735425477_introduction Abstract View Chapter Open the PDF Link PDF for Introduction in another window
- Chapter 1: Sound and Waves By Sergej Faletič Sergej Faletič Faculty of Mathematics and Physics, University of Ljubljana , Ljubljana, Slovenia Search for other works by this author on: This Site PubMed Google Scholar Doi: https://doi.org/10.1063/9780735425477_001 Open the PDF Link PDF for Chapter 1: Sound and Waves in another window
- Chapter 2: Fluids in Equilibrium and Hydrodynamics By Onofrio Rosario Battaglia ; Onofrio Rosario Battaglia Dipartimento di Fisica e Chimica—Emilio Segrè, Università degli Studi di Palermo , 90128, Palermo, Italy Search for other works by this author on: This Site PubMed Google Scholar Giulia Termini ; Giulia Termini Dipartimento di Fisica e Chimica—Emilio Segrè, Università degli Studi di Palermo , 90128, Palermo, Italy Search for other works by this author on: This Site PubMed Google Scholar Claudio Fazio Claudio Fazio Dipartimento di Fisica e Chimica—Emilio Segrè, Università degli Studi di Palermo , 90128, Palermo, Italy Search for other works by this author on: This Site PubMed Google Scholar Doi: https://doi.org/10.1063/9780735425477_002 Open the PDF Link PDF for Chapter 2: Fluids in Equilibrium and Hydrodynamics in another window
- Chapter 3: Student Understanding of Thermal Physics By Michael E. Loverude Michael E. Loverude Department of Physics, California State University Fullerton , Fullerton, California 92831, USA Search for other works by this author on: This Site PubMed Google Scholar Doi: https://doi.org/10.1063/9780735425477_003 Open the PDF Link PDF for Chapter 3: Student Understanding of Thermal Physics in another window
- Chapter 4: Energy By Knut Neumann ; Knut Neumann Leibniz Institute for Science and Mathematics Education (IPN) , 24118 Kiel, Germany Search for other works by this author on: This Site PubMed Google Scholar Jeffrey C. Nordine Jeffrey C. Nordine Leibniz Institute for Science and Mathematics Education (IPN) , 24118 Kiel, Germany University of Iowa , Iowa City, Iowa 52240, USA Search for other works by this author on: This Site PubMed Google Scholar Doi: https://doi.org/10.1063/9780735425477_004 Open the PDF Link PDF for Chapter 4: Energy in another window
- Chapter 5: Students' Understandings of Electricity and Magnetism By Paul van Kampen ; Paul van Kampen Centre for the Advancement of STEM Teaching and Learning & School of Physical Sciences, Dublin City University , Glasnevin, Dublin 9, Ireland Search for other works by this author on: This Site PubMed Google Scholar Mieke De Cock Mieke De Cock Department of Physics and Astronomy & LESEC, KU Leuven, 3001 Leuven, Belgium Search for other works by this author on: This Site PubMed Google Scholar Doi: https://doi.org/10.1063/9780735425477_005 Open the PDF Link PDF for Chapter 5: Students' Understandings of Electricity and Magnetism in another window
- Chapter 6: Teaching and Learning Optics at the Secondary Level By Claudia Haagen-Schützenhöfer ; Claudia Haagen-Schützenhöfer Naturwissenschaftliche Fakultät, Karl Franzens Universität , Graz, Steiermark, Austria Search for other works by this author on: This Site PubMed Google Scholar Thomas Schubatzky ; Thomas Schubatzky Department of Subject-Specific Education, University of Innsbruck, Innsbruck, AustriaInstitute for Experimental Physics, University of Innsbruck , Innsbruck, Austria Search for other works by this author on: This Site PubMed Google Scholar Markus Obczovsky Markus Obczovsky University of Graz , Graz, Austria Search for other works by this author on: This Site PubMed Google Scholar Doi: https://doi.org/10.1063/9780735425477_006 Open the PDF Link PDF for Chapter 6: Teaching and Learning Optics at the Secondary Level in another window
- Chapter 7: Research on Student Learning of Foundational Concepts in Galilean and Relativistic Kinematics: The Role of Operational Definitions By Andrew Boudreaux ; Andrew Boudreaux Department of Physics, Western Washington University , Bellingham, Washington 98225-9164, USA Search for other works by this author on: This Site PubMed Google Scholar Stamatis Vokos ; Stamatis Vokos Physics Department, California Polytechnic State University , San Luis Obispo, California, USA Search for other works by this author on: This Site PubMed Google Scholar Rachel E. Scherr Rachel E. Scherr School of STEM, University of Washington , Bothell, Washington, USA Search for other works by this author on: This Site PubMed Google Scholar Doi: https://doi.org/10.1063/9780735425477_007 Open the PDF Link PDF for Chapter 7: Research on Student Learning of Foundational Concepts in Galilean and Relativistic Kinematics: The Role of Operational Definitions in another window
- Chapter 8: Research Studies on Learning Quantum Physics By Marisa Michelini ; Marisa Michelini Research Institute in Physics Education, University of Udine , Via delle Scienze 206, 33100 Udine, Italy Search for other works by this author on: This Site PubMed Google Scholar Alberto Stefanel Alberto Stefanel Research Unit in Physics Education, University of Udine , Via delle Scienze 206, 33100 Udine, Italy Search for other works by this author on: This Site PubMed Google Scholar Doi: https://doi.org/10.1063/9780735425477_008 Open the PDF Link PDF for Chapter 8: Research Studies on Learning Quantum Physics in another window
- Chapter 9: Radioactivity, Radiation, and Particle Physics in General By Michael M. Hull ; Michael M. Hull Search for other works by this author on: This Site PubMed Google Scholar Alexandra Jansky ; Alexandra Jansky Berner Fachhochschule Architektur , Holz und Bau, Biel, Switzerland Search for other works by this author on: This Site PubMed Google Scholar Martin Hopf Martin Hopf Austrian Educational Competence Centre Physics and Faculty of Physics, University of Vienna , Vienna, Austria Search for other works by this author on: This Site PubMed Google Scholar Doi: https://doi.org/10.1063/9780735425477_009 Open the PDF Link PDF for Chapter 9: Radioactivity, Radiation, and Particle Physics in General in another window
- Chapter 10: Two Perspectives on Physics Problem Solving and Their Relation to Adaptive Expertise By Eric Kuo Eric Kuo University of Illinois Urbana-Champaign , Urbana, Illinois 61801, USA Search for other works by this author on: This Site PubMed Google Scholar Doi: https://doi.org/10.1063/9780735425477_010 Open the PDF Link PDF for Chapter 10: Two Perspectives on Physics Problem Solving and Their Relation to Adaptive Expertise in another window
- Chapter 11: Theorizing Concept Learning in Physics Education Research: Progress and Prospects By Tamer G. Amin ; Tamer G. Amin Department of Education, American University of Beirut , Beirut 1107 2020, Lebanon a) Search for other works by this author on: This Site PubMed Google Scholar Mariana Levin ; Mariana Levin Department of Mathematics, Western Michigan University , Kalamazoo, Michigan 49008, USA a) Search for other works by this author on: This Site PubMed Google Scholar Olivia Levrini Olivia Levrini Department of Physics and Astronomy “A. Righi,” Alma Mater Studiorum–University of Bologna , 40126 Bologna, Italy a) Search for other works by this author on: This Site PubMed Google Scholar Doi: https://doi.org/10.1063/9780735425477_011 Open the PDF Link PDF for Chapter 11: Theorizing Concept Learning in Physics Education Research: Progress and Prospects in another window
- Chapter 12: Epistemic Models of Sensemaking and Reasoning By Shulamit Kapon ; Shulamit Kapon Education in Science and Technology, Technion–Israel Institute of Technology , Haifa 3200003, Israel Search for other works by this author on: This Site PubMed Google Scholar Leema Berland Leema Berland University of Wisconsin–Madison , Madison, Wisconsin 53706, USA Search for other works by this author on: This Site PubMed Google Scholar Doi: https://doi.org/10.1063/9780735425477_012 Open the PDF Link PDF for Chapter 12: Epistemic Models of Sensemaking and Reasoning in another window
- Chapter 13: Learning in Interaction: Interacting Scales of Research By Enrique Suárez ; Enrique Suárez University of Massachusetts , Amherst, USA Search for other works by this author on: This Site PubMed Google Scholar Gina Quan ; Gina Quan San José State University , USA Search for other works by this author on: This Site PubMed Google Scholar David Hammer ; David Hammer Tufts University , USA Search for other works by this author on: This Site PubMed Google Scholar Leslie Atkins Leslie Atkins Boise State University , USA Search for other works by this author on: This Site PubMed Google Scholar Doi: https://doi.org/10.1063/9780735425477_013 Open the PDF Link PDF for Chapter 13: Learning in Interaction: Interacting Scales of Research in another window
- Chapter 14: Affect in Physics Learning: Entanglement with Cognition and Learning Goals By Lama Z. Jaber ; Lama Z. Jaber Florida State University , USA Search for other works by this author on: This Site PubMed Google Scholar Leslie Atkins ; Leslie Atkins Boise State University , USA Search for other works by this author on: This Site PubMed Google Scholar Andrew Elby ; Andrew Elby University of Maryland , USA Search for other works by this author on: This Site PubMed Google Scholar Enrique Suárez Enrique Suárez University of Massachusetts , Amherst, USA Search for other works by this author on: This Site PubMed Google Scholar Doi: https://doi.org/10.1063/9780735425477_014 Open the PDF Link PDF for Chapter 14: Affect in Physics Learning: Entanglement with Cognition and Learning Goals in another window
- Chapter 15: PER Informed “Physics Teaching”—Coordinating Learning Goals and Teaching Practices By Edit Yerushalmi ; Edit Yerushalmi Weizmann Institute of Science , Israel Search for other works by this author on: This Site PubMed Google Scholar Bat Sheva Eylon Bat Sheva Eylon Weizmann Institute of Science , Israel Search for other works by this author on: This Site PubMed Google Scholar Doi: https://doi.org/10.1063/9780735425477_015 Open the PDF Link PDF for Chapter 15: PER Informed “Physics Teaching”—Coordinating Learning Goals and Teaching Practices in another window
- Chapter 16: Argumentation in Physics Education Research: Recent Trends and Key Themes By Sibel Erduran ; Sibel Erduran University of Oxford , Oxford, UK Search for other works by this author on: This Site PubMed Google Scholar Wonyong Park Wonyong Park University of Southampton , Southampton, UK Search for other works by this author on: This Site PubMed Google Scholar Doi: https://doi.org/10.1063/9780735425477_016 Open the PDF Link PDF for Chapter 16: Argumentation in Physics Education Research: Recent Trends and Key Themes in another window
- Chapter 17: Instructional Strategies that Foster Effective Problem-Solving By Chandralekha Singh ; Chandralekha Singh Department of Physics and Astronomy, University of Pittsburgh , Pittsburgh, PA, USA Search for other works by this author on: This Site PubMed Google Scholar Alexandru Maries ; Alexandru Maries Department of Physics, University of Cincinnati , Cincinnati OH, USA Search for other works by this author on: This Site PubMed Google Scholar Kenneth Heller ; Kenneth Heller School of Physics and Astronomy, University of Minnesota , Minneapolis, MN, USA Search for other works by this author on: This Site PubMed Google Scholar Patricia Heller Patricia Heller Department of Curriculum and Instruction, University of Minnesota , Minneapolis, MN, USA Search for other works by this author on: This Site PubMed Google Scholar Doi: https://doi.org/10.1063/9780735425477_017 Open the PDF Link PDF for Chapter 17: Instructional Strategies that Foster Effective Problem-Solving in another window
- Chapter 18: Instructional Strategies that Foster Experimental Physics Skills By Natasha G. Holmes ; Natasha G. Holmes Laboratory of Atomic and Solid State Physics, Cornell University , Ithaca, NY, USA Search for other works by this author on: This Site PubMed Google Scholar Emily M. Smith Emily M. Smith Department of Physics, Colorado School of Mines , Golden, CO, USA Search for other works by this author on: This Site PubMed Google Scholar Doi: https://doi.org/10.1063/9780735425477_018 Open the PDF Link PDF for Chapter 18: Instructional Strategies that Foster Experimental Physics Skills in another window
- Chapter 19: Physics Computational Literacy: What, Why, and How? By Tor Ole B. Odden ; Tor Ole B. Odden Department of Physics, University of Oslo , Oslo Norway Search for other works by this author on: This Site PubMed Google Scholar Marcos D. Caballero Marcos D. Caballero Department of Physics, University of Oslo , Oslo Norway Department of Computational Mathematics, Science, and Engineering, CREATE for STEM Institute, Michigan State University , East Lansing, MI, USA Search for other works by this author on: This Site PubMed Google Scholar Doi: https://doi.org/10.1063/9780735425477_019 Open the PDF Link PDF for Chapter 19: Physics Computational Literacy: What, Why, and How? in another window
- Chapter 20: Introductory Physics for Life Sciences: Preparing and Engaging Students through Authentic Interdisciplinary Connections By Catherine H. Crouch ; Catherine H. Crouch Swarthmore College , USA Search for other works by this author on: This Site PubMed Google Scholar Benjamin Geller Benjamin Geller Swarthmore College , USA Search for other works by this author on: This Site PubMed Google Scholar Doi: https://doi.org/10.1063/9780735425477_020 Open the PDF Link PDF for Chapter 20: Introductory Physics for Life Sciences: Preparing and Engaging Students through Authentic Interdisciplinary Connections in another window
- Chapter 21: Physics in K-12 Integrated Science Curricula By Elon Langbeheim ; Elon Langbeheim Ben-Gurion University of the Negev , Negev, Israel Search for other works by this author on: This Site PubMed Google Scholar Yaron Lehavi ; Yaron Lehavi The David Yellin Academic College of Education , Israel Search for other works by this author on: This Site PubMed Google Scholar Avraham Merzel Avraham Merzel The Hebrew University of Jerusalem , Jerusalem, Israel Search for other works by this author on: This Site PubMed Google Scholar Doi: https://doi.org/10.1063/9780735425477_021 Open the PDF Link PDF for Chapter 21: Physics in K-12 Integrated Science Curricula in another window
- Chapter 22: The Interplay between Coherent Instruction and Learning Progressions By Jeffrey C. Nordine ; Jeffrey C. Nordine University of Iowa , Iowa City, Iowa, USA Leibniz Institute for Science and Mathematics Education (IPN) , Kiel, Germany Search for other works by this author on: This Site PubMed Google Scholar Knut Neumann Knut Neumann Leibniz Institute for Science and Mathematics Education (IPN) , Kiel, Germany Search for other works by this author on: This Site PubMed Google Scholar Doi: https://doi.org/10.1063/9780735425477_022 Open the PDF Link PDF for Chapter 22: The Interplay between Coherent Instruction and Learning Progressions in another window
- Chapter 23: Physics Education Research and the Development of Active Learning Strategies in Introductory Physics By David R. Sokoloff ; David R. Sokoloff University of Oregon , Eugene, Oregon 97405, USA Search for other works by this author on: This Site PubMed Google Scholar Tuğba Yüksel Tuğba Yüksel Department of Mathematics and Science Education, College of Education, Recep Tayyip Erdoğan University , Turkey Search for other works by this author on: This Site PubMed Google Scholar Doi: https://doi.org/10.1063/9780735425477_023 Open the PDF Link PDF for Chapter 23: Physics Education Research and the Development of Active Learning Strategies in Introductory Physics in another window
- Chapter 24: Teaching Practices By Andrée Tiberghien ; Andrée Tiberghien UMR ICAR, University of Lyon , France Search for other works by this author on: This Site PubMed Google Scholar Patrice Venturini Patrice Venturini UMR EFTS, University of Toulouse , France Search for other works by this author on: This Site PubMed Google Scholar Doi: https://doi.org/10.1063/9780735425477_024 Open the PDF Link PDF for Chapter 24: Teaching Practices in another window
- Index By Mehmet Fatih Taşar ; Mehmet Fatih Taşar Georgia State University , Atlanta, GA, USA Search for other works by this author on: This Site PubMed Google Scholar Paula R. L. Heron Paula R. L. Heron University of Washington , Seattle, WA, USA Search for other works by this author on: This Site PubMed Google Scholar Doi: https://doi.org/10.1063/9780735425477_index Abstract View Chapter Open the PDF Link PDF for Index in another window
- For Researchers
- For Librarians
- For Advertisers
- Our Publishing Partners
- Physics Today
- Conference Proceedings
- Special Topics
pubs.aip.org
- Privacy Policy
- Terms of Use
Connect with AIP Publishing
This feature is available to subscribers only.
Sign In or Create an Account
- Position paper
- Open access
- Published: 28 November 2019
Physics education research for 21 st century learning
- Lei Bao ORCID: orcid.org/0000-0003-3348-4198 1 &
- Kathleen Koenig 2
Disciplinary and Interdisciplinary Science Education Research volume 1 , Article number: 2 ( 2019 ) Cite this article
38k Accesses
74 Citations
2 Altmetric
Metrics details
Education goals have evolved to emphasize student acquisition of the knowledge and attributes necessary to successfully contribute to the workforce and global economy of the twenty-first Century. The new education standards emphasize higher end skills including reasoning, creativity, and open problem solving. Although there is substantial research evidence and consensus around identifying essential twenty-first Century skills, there is a lack of research that focuses on how the related subskills interact and develop over time. This paper provides a brief review of physics education research as a means for providing a context towards future work in promoting deep learning and fostering abilities in high-end reasoning. Through a synthesis of the literature around twenty-first Century skills and physics education, a set of concretely defined education and research goals are suggested for future research, along with how these may impact the next generation physics courses and how physics should be taught in the future.
Introduction
Education is the primary service offered by society to prepare its future generation workforce. The goals of education should therefore meet the demands of the changing world. The concept of learner-centered, active learning has broad, growing support in the research literature as an empirically validated teaching practice that best promotes learning for modern day students (Freeman et al., 2014 ). It stems out of the constructivist view of learning, which emphasizes that it is the learner who needs to actively construct knowledge and the teacher should assume the role of a facilitator rather than the source of knowledge. As implied by the constructivist view, learner-centered education usually emphasizes active-engagement and inquiry style teaching-learning methods, in which the learners can effectively construct their understanding under the guidance of instruction. The learner-centered education also requires educators and researchers to focus their efforts on the learners’ needs, not only to deliver effective teaching-learning approaches, but also to continuously align instructional practices to the education goals of the times. The goals of introductory college courses in science, technology, engineering, and mathematics (STEM) disciplines have constantly evolved from some notion of weed-out courses that emphasize content drilling, to the current constructivist active-engagement type of learning that promotes interest in STEM careers and fosters high-end cognitive abilities.
Following the conceptually defined framework of twenty-first Century teaching and learning, this paper aims to provide contextualized operational definitions of the goals for twenty-first Century learning in physics (and STEM in general) as well as the rationale for the importance of these outcomes for current students. Aligning to the twenty-first Century learning goals, research in physics education is briefly reviewed to provide a context towards future work in promoting deep learning and fostering abilities in high-end reasoning in parallel. Through a synthesis of the literature around twenty-first Century skills and physics education, a set of concretely defined education and research goals are suggested for future research. These goals include: domain-specific research in physics learning; fostering scientific reasoning abilities that are transferable across the STEM disciplines; and dissemination of research-validated curriculum and approaches to teaching and learning. Although this review has a focus on physics education research (PER), it is beneficial to expand the perspective to view physics education in the broader context of STEM learning. Therefore, much of the discussion will blend PER with STEM education as a continuum body of work on teaching and learning.
Education goals for twenty-first century learning
Education goals have evolved to emphasize student acquisition of essential “21 st Century skills”, which define the knowledge and attributes necessary to successfully contribute to the workforce and global economy of the 21st Century (National Research Council, 2011 , 2012a ). In general, these standards seek to transition from emphasizing content-based drilling and memorization towards fostering higher-end skills including reasoning, creativity, and open problem solving (United States Chamber of Commerce, 2017 ). Initiatives on advancing twenty-first Century education focus on skills that converge on three broad clusters: cognitive, interpersonal, and intrapersonal, all of which include a rich set of sub-dimensions.
Within the cognitive domain, multiple competencies have been proposed, including deep learning, non-routine problem solving, systems thinking, critical thinking, computational and information literacy, reasoning and argumentation, and innovation (National Research Council, 2012b ; National Science and Technology Council, 2018 ). Interpersonal skills are those necessary for relating to others, including the ability to work creatively and collaboratively as well as communicate clearly. Intrapersonal skills, on the other hand, reside within the individual and include metacognitive thinking, adaptability, and self-management. These involve the ability to adjust one’s strategy or approach along with the ability to work towards important goals without significant distraction, both essential for sustained success in long-term problem solving and career development.
Although many descriptions exist for what qualifies as twenty-first Century skills, student abilities in scientific reasoning and critical thinking are the most commonly noted and widely studied. They are highly connected with the other cognitive skills of problem solving, decision making, and creative thinking (Bailin, 1996 ; Facione, 1990 ; Fisher, 2001 ; Lipman, 2003 ; Marzano et al., 1988 ), and have been important educational goals since the 1980s (Binkley et al., 2010 ; NCET, 1987 ). As a result, they play a foundational role in defining, assessing, and developing twenty-first Century skills.
The literature for critical thinking is extensive (Bangert-Drowns & Bankert, 1990 ; Facione, 1990 ; Glaser, 1941 ). Various definitions exist with common underlying principles. Broadly defined, critical thinking is the application of the cognitive skills and strategies that aim for and support evidence-based decision making. It is the thinking involved in solving problems, formulating inferences, calculating likelihoods, and making decisions (Halpern, 1999 ). It is the “reasonable reflective thinking focused on deciding what to believe or do” (Ennis, 1993 ). Critical thinking is recognized as a way to understand and evaluate subject matter; producing reliable knowledge and improving thinking itself (Paul, 1990 ; Siegel, 1988 ).
The notion of scientific reasoning is often used to label the set of skills that support critical thinking, problem solving, and creativity in STEM. Broadly defined, scientific reasoning includes the thinking and reasoning skills involved in inquiry, experimentation, evidence evaluation, inference and argument that support the formation and modification of concepts and theories about the natural world; such as the ability to systematically explore a problem, formulate and test hypotheses, manipulate and isolate variables, and observe and evaluate consequences (Bao et al., 2009 ; Zimmerman, 2000 ). Critical thinking and scientific reasoning share many features, where both emphasize evidence-based decision making in multivariable causal conditions. Critical thinking can be promoted through the development of scientific reasoning, which includes student ability to reach a reliable conclusion after identifying a question, formulating hypotheses, gathering relevant data, and logically testing and evaluating the hypothesis. In this way, scientific reasoning can be viewed as a scientific domain instantiation of critical thinking in the context of STEM learning.
In STEM learning, cognitive aspects of the twenty-first Century skills aim to develop reasoning skills, critical thinking skills, and deep understanding, all of which allow students to develop well connected expert-like knowledge structures and engage in meaningful scientific inquiry and problem solving. Within physics education, a core component of STEM education, the learning of conceptual understanding and problem solving remains a current emphasis. However, the fast-changing work environment and technology-driven world require a new set of core knowledge, skills, and habits of mind to solve complex interdisciplinary problems, gather and evaluate evidence, and make sense of information from a variety of sources (Tanenbaum, 2016 ). The education goals in physics are transitioning towards ability fostering as well as extension and integration with other STEM disciplines. Although curriculum that supports these goals is limited, there are a number of attempts, particularly in developing active learning classrooms and inquiry-based laboratory activities, which have demonstrated success. Some of these are described later in this paper as they provide a foundation for future work in physics education.
Interpersonal skills, such as communication and collaboration, are also essential for twenty-first Century problem-solving tasks, which are often open-ended, complex, and team-based. As the world becomes more connected in a multitude of dimensions, tackling significant problems involving complex systems often goes beyond the individual and requires working with others who are increasingly from culturally diverse backgrounds. Due to the rise of communication technologies, being able to articulate thoughts and ideas in a variety of formats and contexts is crucial, as well as the ability to effectively listen or observe to decipher meaning. Interpersonal skills can be promoted by integrating group-learning experiences into the classroom setting, while providing students with the opportunity to engage in open-ended tasks with a team of peer learners who may propose more than one plausible solution. These experiences should be designed such that students must work collaboratively and responsibly in teams to develop creative solutions, which are later disseminated through informative presentations and clearly written scientific reports. Although educational settings in general have moved to providing students with more and more opportunities for collaborative learning, a lack of effective assessments for these important skills has been a limiting factor for producing informative research and widespread implementation. See Liu ( 2010 ) for an overview of measurement instruments reported in the research literature.
Intrapersonal skills are based on the individual and include the ability to manage one’s behavior and emotions to achieve goals. These are especially important for adapting in the fast-evolving collaborative modern work environment and for learning new tasks to solve increasingly challenging interdisciplinary problems, both of which require intellectual openness, work ethic, initiative, and metacognition, to name a few. These skills can be promoted using instruction which, for example, includes metacognitive learning strategies, provides opportunities to make choices and set goals for learning, and explicitly connects to everyday life events. However, like interpersonal skills, the availability of relevant assessments challenges advancement in this area. In this review, the vast amount of studies on interpersonal and intrapersonal skills will not be discussed in order to keep the main focus on the cognitive side of skills and reasoning.
The purpose behind discussing twenty-first Century skills is that this set of skills provides important guidance for establishing essential education goals for modern society and learners. However, although there is substantial research evidence and consensus around identifying necessary twenty-first Century skills, there is a lack of research that focuses on how the related subskills interact and develop over time (Reimers & Chung, 2016 ), with much of the existing research residing in academic literature that is focused on psychology rather than education systems (National Research Council, 2012a ). Therefore, a major and challenging task for discipline-based education researchers and educators is to operationally define discipline-specific goals that align with the twenty-first Century skills for each of the STEM fields. In the following sections, this paper will provide a limited vision of the research endeavors in physics education that can translate the past and current success into sustained impact for twenty-first Century teaching and learning.
Proposed education and research goals
Physics education research (PER) is often considered an early pioneer in discipline-based education research (National Research Council, 2012c ), with well-established, broad, and influential outcomes (e.g., Hake, 1998 ; Hsu, Brewe, Foster, & Harper, 2004 ; McDermott & Redish, 1999 ; Meltzer & Thornton, 2012 ). Through the integration of twenty-first Century skills with the PER literature, a set of broadly defined education and research goals is proposed for future PER work:
Discipline-specific deep learning: Cognitive and education research involving physics learning has established a rich literature on student learning behaviors along with a number of frameworks. Some of the popular frameworks include conceptual understanding and concept change, problem solving, knowledge structure, deep learning, and knowledge integration. Aligned with twenty-first Century skills, future research in physics learning should aim to integrate the multiple areas of existing work, such that they help students develop well integrated knowledge structures in order to achieve deep leaning in physics.
Fostering scientific reasoning for transfer across STEM disciplines: The broad literature in physics learning and scientific reasoning can provide a solid foundation to further develop effective physics education approaches, such as active engagement instruction and inquiry labs, specifically targeting scientific inquiry abilities and reasoning skills. Since scientific reasoning is a more domain-general cognitive ability, success in physics can also more readily inform research and education practices in other STEM fields.
Research, development, assessment, and dissemination of effective education approaches: Developing and maintaining a supportive infrastructure of education research and implementation has always been a challenge, not only in physics but in all STEM areas. The twenty-first Century education requires researchers and instructors across STEM to work together as an extended community in order to construct a sustainable integrated STEM education environment. Through this new infrastructure, effective team-based inquiry learning and meaningful assessment can be delivered to help students develop a comprehensive skills set including deep understanding and scientific reasoning, as well as communication and other non-cognitive abilities.
The suggested research will generate understanding and resources to support education practices that meet the requirements of the Next Generation Science Standards (NGSS), which explicitly emphasize three areas of learning including disciplinary core ideas, crosscutting concepts, and practices (National Research Council, 2012b ). The first goal for promoting deep learning of disciplinary knowledge corresponds well to the NGSS emphasis on disciplinary core ideas, which play a central role in helping students develop well integrated knowledge structures to achieve deep understanding. The second goal on fostering transferable scientific reasoning skills supports the NGSS emphasis on crosscutting concepts and practices. Scientific reasoning skills are crosscutting cognitive abilities that are essential to the development of domain-general concepts and modeling strategies. In addition, the development of scientific reasoning requires inquiry-based learning and practices. Therefore, research on scientific reasoning can produce a valuable knowledge base on education means that are effective for developing crosscutting concepts and promoting meaningful practices in STEM. The third research goal addresses the challenge in the assessment of high-end skills and the dissemination of effective educational approaches, which supports all NGSS initiatives to ensure sustainable development and lasting impact. The following sections will discuss the research literature that provides the foundation for these three research goals and identify the specific challenges that will need to be addressed in future work.
Promoting deep learning in physics education
Physics education for the twenty-first Century aims to foster high-end reasoning skills and promote deep conceptual understanding. However, many traditional education systems place strong emphasis on only problem solving with the expectation that students obtain deep conceptual understanding through repetitive problem-solving practices, which often doesn’t occur (Alonso, 1992 ). This focus on problem solving has been shown to have limitations as a number of studies have revealed disconnections between learning conceptual understanding and problem-solving skills (Chiu, 2001 ; Chiu, Guo, & Treagust, 2007 ; Hoellwarth, Moelter, & Knight, 2005 ; Kim & Pak, 2002 ; Nakhleh, 1993 ; Nakhleh & Mitchell, 1993 ; Nurrenbern & Pickering, 1987 ; Stamovlasis, Tsaparlis, Kamilatos, Papaoikonomou, & Zarotiadou, 2005 ). In fact, drilling in problem solving may actually promote memorization of context-specific solutions with minimal generalization rather than transitioning students from novices to experts.
Towards conceptual understanding and learning, many models and definitions have been established to study and describe student conceptual knowledge states and development. For example, students coming into a physics classroom often hold deeply rooted, stable understandings that differ from expert conceptions. These are commonly referred to as misconceptions or alternative conceptions (Clement, 1982 ; Duit & Treagust, 2003 ; Dykstra Jr, Boyle, & Monarch, 1992 ; Halloun & Hestenes, 1985a , 1985b ). Such students’ conceptions are context dependent and exist as disconnected knowledge fragments, which are strongly situated within specific contexts (Bao & Redish, 2001 , 2006 ; Minstrell, 1992 ).
In modeling students’ knowledge structures, DiSessa’s proposed phenomenological primitives (p-prim) describe a learner’s implicit thinking, cued from specific contexts, as an underpinning cognitive construct for a learner’s expressed conception (DiSessa, 1993 ; Smith III, DiSessa, & Roschelle, 1994 ). Facets, on the other hand, map between the implicit p-prim and concrete statements of beliefs and are developed as discrete and independent units of thought, knowledge, or strategies used by individuals to address specific situations (Minstrell, 1992 ). Ontological categories, defined by Chi, describe student reasoning in the most general sense. Chi believed that these are distinct, stable, and constraining, and that a core reason behind novices’ difficulties in physics is that they think of physics within the category of matter instead of processes (Chi, 1992 ; Chi & Slotta, 1993 ; Chi, Slotta, & De Leeuw, 1994 ; Slotta, Chi, & Joram, 1995 ). More details on conceptual learning and problem solving are well summarized in the literature (Hsu et al., 2004 ; McDermott & Redish, 1999 ), from which a common theme emerges from the models and definitions. That is, learning is context dependent and students with poor conceptual understanding typically have locally connected knowledge structures with isolated conceptual constructs that are unable to establish similarities and contrasts between contexts.
Additionally, this idea of fragmentation is demonstrated through many studies on student problem solving in physics and other fields. It has been shown that a student’s knowledge organization is a key aspect for distinguishing experts from novices (Bagno, Eylon, & Ganiel, 2000 ; Chi, Feltovich, & Glaser, 1981 ; De Jong & Ferguson-Hesler, 1986 ; Eylon & Reif, 1984 ; Ferguson-Hesler & De Jong, 1990 ; Heller & Reif, 1984 ; Larkin, McDermott, Simon, & Simon, 1980 ; Smith, 1992 ; Veldhuis, 1990 ; Wexler, 1982 ). Expert’s knowledge is organized around core principles of physics, which are applied to guide problem solving and develop connections between different domains as well as new, unfamiliar situations (Brown, 1989 ; Perkins & Salomon, 1989 ; Salomon & Perkins, 1989 ). Novices, on the other hand, lack a well-organized knowledge structure and often solve problems by relying on surface features that are directly mapped to certain problem-solving outcomes through memorization (Chi, Bassok, Lewis, Reimann, & Glaser, 1989 ; Hardiman, Dufresne, & Mestre, 1989 ; Schoenfeld & Herrmann, 1982 ).
This lack of organization creates many difficulties in the comprehension of basic concepts and in solving complex problems. This leads to the common complaint that students’ knowledge of physics is reduced to formulas and vague labels of the concepts, which are unable to substantively contribute to meaningful reasoning processes. A novice’s fragmented knowledge structure severely limits the learner’s conceptual understanding. In essence, these students are able to memorize how to approach a problem given specific information but lack the understanding of the underlying concept of the approach, limiting their ability to apply this approach to a novel situation. In order to achieve expert-like understanding, a student’s knowledge structure must integrate all of the fragmented ideas around the core principle to form a coherent and fully connected conceptual framework.
Towards a more general theoretical consideration, students’ alternative conceptions and fragmentation in knowledge structures can be viewed through both the “naïve theory” framework (e.g., Posner, Strike, Hewson, & Gertzog, 1982 ; Vosniadou, Vamvakoussi, & Skopeliti, 2008 ) and the “knowledge in pieces” (DiSessa, 1993 ) perspective. The “naïve theory” framework considers students entering the classroom with stable and coherent ideas (naïve theories) about the natural world that differ from those presented by experts. In the “knowledge in pieces” perspective, student knowledge is constructed in real-time and incorporates context features with the p-prims to form the observed conceptual expressions. Although there exists an ongoing debate between these two views (Kalman & Lattery, 2018 ), it is more productive to focus on their instructional implications for promoting meaningful conceptual change in students’ knowledge structures.
In the process of learning, students may enter the classroom with a range of initial states depending on the population and content. For topics with well-established empirical experiences, students often have developed their own ideas and understanding, while on topics without prior exposure, students may create their initial understanding in real-time based on related prior knowledge and given contextual features (Bao & Redish, 2006 ). These initial states of understanding, regardless of their origin, are usually different from those of experts. Therefore, the main function of teaching and learning is to guide students to modify their initial understanding towards the experts’ views. Although students’ initial understanding may exist as a body of coherent ideas within limited contexts, as students start to change their knowledge structures throughout the learning process, they may evolve into a wide range of transitional states with varying levels of knowledge integration and coherence. The discussion in this brief review on students’ knowledge structures regarding fragmentation and integration are primarily focused on the transitional stages emerged through learning.
The corresponding instructional goal is then to help students more effectively develop an integrated knowledge structure so as to achieve a deep conceptual understanding. From an educator’s perspective, Bloom’s taxonomy of education objectives establishes a hierarchy of six levels of cognitive skills based on their specificity and complexity: Remember (lowest and most specific), Understand, Apply, Analyze, Evaluate, and Create (highest and most general and complex) (Anderson et al., 2001 ; Bloom, Engelhart, Furst, Hill, & Krathwohl, 1956 ). This hierarchy of skills exemplifies the transition of a learner’s cognitive development from a fragmented and contextually situated knowledge structure (novice with low level cognitive skills) to a well-integrated and globally networked expert-like structure (with high level cognitive skills).
As a student’s learning progresses from lower to higher cognitive levels, the student’s knowledge structure becomes more integrated and is easier to transfer across contexts (less context specific). For example, beginning stage students may only be able to memorize and perform limited applications of the features of certain contexts and their conditional variations, with which the students were specifically taught. This leads to the establishment of a locally connected knowledge construct. When a student’s learning progresses from the level of Remember to Understand, the student begins to develop connections among some of the fragmented pieces to form a more fully connected network linking a larger set of contexts, thus advancing into a higher level of understanding. These connections and the ability to transfer between different situations form the basis of deep conceptual understanding. This growth of connections leads to a more complete and integrated cognitive structure, which can be mapped to a higher level on Bloom’s taxonomy. This occurs when students are able to relate a larger number of different contextual and conditional aspects of a concept for analyzing and evaluating to a wider variety of problem situations.
Promoting the growth of connections would appear to aid in student learning. Exactly which teaching methods best facilitate this are dependent on the concepts and skills being learned and should be determined through research. However, it has been well recognized that traditional instruction often fails to help students obtain expert-like conceptual understanding, with many misconceptions still existing after instruction, indicating weak integration within a student’s knowledge structure (McKeachie, 1986 ).
Recognizing the failures of traditional teaching, various research-informed teaching methods have been developed to enhance student conceptual learning along with diagnostic tests, which aim to measure the existence of misconceptions. Most advances in teaching methods focus on the inclusion of inquiry-based interactive-engagement elements in lecture, recitations, and labs. In physics education, these methods were popularized after Hake’s landmark study demonstrated the effectiveness of interactive-engagement over traditional lectures (Hake, 1998 ). Some of these methods include the use of peer instruction (Mazur, 1997 ), personal response systems (e.g., Reay, Bao, Li, Warnakulasooriya, & Baugh, 2005 ), studio-style instruction (Beichner et al., 2007 ), and inquiry-based learning (Etkina & Van Heuvelen, 2001 ; Laws, 2004 ; McDermott, 1996 ; Thornton & Sokoloff, 1998 ). The key approach of these methods aims to improve student learning by carefully targeting deficits in student knowledge and actively encouraging students to explore and discuss. Rather than rote memorization, these approaches help promote generalization and deeper conceptual understanding by building connections between knowledge elements.
Based on the literature, including Bloom’s taxonomy and the new education standards that emphasize twenty-first Century skills, a common focus on teaching and learning can be identified. This focus emphasizes helping students develop connections among fragmented segments of their knowledge pieces and is aligned with the knowledge integration perspective, which focuses on helping students develop and refine their knowledge structure toward a more coherently organized and extensively connected network of ideas (Lee, Liu, & Linn, 2011 ; Linn, 2005 ; Nordine, Krajcik, & Fortus, 2011 ; Shen, Liu, & Chang, 2017 ). For meaningful learning to occur, new concepts must be integrated into a learner’s existing knowledge structure by linking the new knowledge to already understood concepts.
Forming an integrated knowledge structure is therefore essential to achieving deep learning, not only in physics but also in all STEM fields. However, defining what connections must occur at different stages of learning, as well as understanding the instructional methods necessary for effectively developing such connections within each STEM disciplinary context, are necessary for current and future research. Together these will provide the much needed foundational knowledge base to guide the development of the next generation of curriculum and classroom environment designed around twenty-first Century learning.
Developing scientific reasoning with inquiry labs
Scientific reasoning is part of the widely emphasized cognitive strand of twenty-first Century skills. Through development of scientific reasoning skills, students’ critical thinking, open-ended problem-solving abilities, and decision-making skills can be improved. In this way, targeting scientific reasoning as a curricular objective is aligned with the goals emphasized in twenty-first Century education. Also, there is a growing body of research on the importance of student development of scientific reasoning, which have been found to positively correlate with course achievement (Cavallo, Rozman, Blickenstaff, & Walker, 2003 ; Johnson & Lawson, 1998 ), improvement on concept tests (Coletta & Phillips, 2005 ; She & Liao, 2010 ), engagement in higher levels of problem solving (Cracolice, Deming, & Ehlert, 2008 ; Fabby & Koenig, 2013 ); and success on transfer (Ates & Cataloglu, 2007 ; Jensen & Lawson, 2011 ).
Unfortunately, research has shown that college students are lacking in scientific reasoning. Lawson ( 1992 ) found that ~ 50% of intro biology students are not capable of applying scientific reasoning in learning, including the ability to develop hypotheses, control variables, and design experiments; all necessary for meaningful scientific inquiry. Research has also found that traditional courses do not significantly develop these abilities, with pre-to-post-test gains of 1%–2%, while inquiry-based courses have gains around 7% (Koenig, Schen, & Bao, 2012 ; Koenig, Schen, Edwards, & Bao, 2012 ). Others found that undergraduates have difficulty developing evidence-based decisions and differentiating between and linking evidence with claims (Kuhn, 1992 ; Shaw, 1996 ; Zeineddin & Abd-El-Khalick, 2010 ). A large scale international study suggested that learning of physics content knowledge with traditional teaching practices does not improve students’ scientific reasoning skills (Bao et al., 2009 ).
Aligned to twenty-first Century learning, it is important to implement curriculum that is specifically designed for developing scientific reasoning abilities within current education settings. Although traditional lectures may continue for decades due to infrastructure constraints, a unique opportunity can be found in the lab curriculum, which may be more readily transformed to include hands-on minds-on group learning activities that are ideal for developing students’ abilities in scientific inquiry and reasoning.
For well over a century, the laboratory has held a distinctive role in student learning (Meltzer & Otero, 2015 ). However, many existing labs, which haven’t changed much since the late 1980s, have received criticism for their outdated cookbook style that lacks effectiveness in developing high-end skills. In addition, labs have been primarily used as a means for verifying the physical principles presented in lecture, and unfortunately, Hofstein and Lunetta ( 1982 ) found in an early review of the literature that research was unable to demonstrate the impact of the lab on student content learning.
About this same time, a shift towards a constructivist view of learning gained popularity and influenced lab curriculum development towards engaging students in the process of constructing knowledge through science inquiry. Curricula, such as Physics by Inquiry (McDermott, 1996 ), Real-Time Physics (Sokoloff, Thornton, & Laws, 2011 ), and Workshop Physics (Laws, 2004 ), were developed with a primary focus on engaging students in cognitive conflict to address misconceptions. Although these approaches have been shown to be highly successful in improving deep learning of physics concepts (McDermott & Redish, 1999 ), the emphasis on conceptual learning does not sufficiently impact the domain general scientific reasoning skills necessitated in the goals of twenty-first Century learning.
Reform in science education, both in terms of targeted content and skills, along with the emergence of knowledge regarding human cognition and learning (Bransford, Brown, & Cocking, 2000 ), have generated renewed interest in the potential of inquiry-based lab settings for skill development. In these types of hands-on minds-on learning, students apply the methods and procedures of science inquiry to investigate phenomena and construct scientific claims, solve problems, and communicate outcomes, which holds promise for developing both conceptual understanding and scientific reasoning skills in parallel (Trowbridge, Bybee, & Powell, 2000 ). In addition, the availability of technology to enhance inquiry-based learning has seen exponential growth, along with the emergence of more appropriate research methodologies to support research on student learning.
Although inquiry-based labs hold promise for developing students’ high-end reasoning, analytic, and scientific inquiry abilities, these educational endeavors have not become widespread, with many existing physics laboratory courses still viewed merely as a place to illustrate the physical principles from the lecture course (Meltzer & Otero, 2015 ). Developing scientific ideas from practical experiences, however, is a complex process. Students need sufficient time and opportunity for interaction and reflection on complex, investigative tasks. Blended learning, which merges lecture and lab (such as studio style courses), addresses this issue to some extent, but has experienced limited adoption, likely due to the demanding infrastructure resources, including dedicated technology-intensive classroom space, equipment and maintenance costs, and fully committed trained staff.
Therefore, there is an immediate need to transform the existing standalone lab courses, within the constraints of the existing education infrastructure, into more inquiry-based designs, with one of its primary goals dedicated to developing scientific reasoning skills. These labs should center on constructing knowledge, along with hands-on minds-on practical skills and scientific reasoning, to support modeling a problem, designing and implementing experiments, analyzing and interpreting data, drawing and evaluating conclusions, and effective communication. In particular, training on scientific reasoning needs to be explicitly addressed in the lab curriculum, which should contain components specifically targeting a set of operationally-defined scientific reasoning skills, such as ability to control variables or engage in multivariate causal reasoning. Although effective inquiry may also implicitly develop some aspects of scientific reasoning skills, such development is far less efficient and varies with context when the primary focus is on conceptual learning.
Several recent efforts to enhance the standalone lab course have shown promise in supporting education goals that better align with twenty-first Century learning. For example, the Investigative Science Learning Environment (ISLE) labs involve a series of tasks designed to help students develop the “habits of mind” of scientists and engineers (Etkina et al., 2006 ). The curriculum targets reasoning as well as the lab learning outcomes published by the American Association of Physics Teachers (Kozminski et al., 2014 ). Operationally, ISLE methods focus on scaffolding students’ developing conceptual understanding using inquiry learning without a heavy emphasis on cognitive conflict, making it more appropriate and effective for entry level students and K-12 teachers.
Likewise, Koenig, Wood, Bortner, and Bao ( 2019 ) have developed a lab curriculum that is intentionally designed around the twenty-first Century learning goals for developing cognitive, interpersonal, and intrapersonal abilities. In terms of the cognitive domain, the lab learning outcomes center on critical thinking and scientific reasoning but do so through operationally defined sub-skills, all of which are transferrable across STEM. These selected sub-skills are found in the research literature, and include the ability to control variables and engage in data analytics and causal reasoning. For each targeted sub-skill, a series of pre-lab and in-class activities provide students with repeated, deliberate practice within multiple hypothetical science-based scenarios followed by real inquiry-based lab contexts. This explicit instructional strategy has been shown to be essential for the development of scientific reasoning (Chen & Klahr, 1999 ). In addition, the Karplus Learning Cycle (Karplus, 1964 ) provides the foundation for the structure of the lab activities and involves cycles of exploration, concept introduction, and concept application. The curricular framework is such that as the course progresses, the students engage in increasingly complex tasks, which allow students the opportunity to learn gradually through a progression from simple to complex skills.
As part of this same curriculum, students’ interpersonal skills are developed, in part, through teamwork, as students work in groups of 3 or 4 to address open-ended research questions, such as, What impacts the period of a pendulum? In addition, due to time constraints, students learn early on about the importance of working together in an efficient manor towards a common goal, with one set of written lab records per team submitted after each lab. Checkpoints built into all in-class activities involve Socratic dialogue between the instructor and students and promote oral communication. This use of directed questioning guides students in articulating their reasoning behind decisions and claims made, while supporting the development of scientific reasoning and conceptual understanding in parallel (Hake, 1992 ). Students’ intrapersonal skills, as well as communication skills, are promoted through the submission of individual lab reports. These reports require students to reflect upon their learning over each of four multi-week experiments and synthesize their ideas into evidence-based arguments, which support a claim. Due to the length of several weeks over which students collect data for each of these reports, the ability to organize the data and manage their time becomes essential.
Despite the growing emphasis on research and development of curriculum that targets twenty-first Century learning, converting a traditionally taught lab course into a meaningful inquiry-based learning environment is challenging in current reform efforts. Typically, the biggest challenge is a lack of resources; including faculty time to create or adapt inquiry-based materials for the local setting, training faculty and graduate student instructors who are likely unfamiliar with this approach, and the potential cost of new equipment. Koenig et al. ( 2019 ) addressed these potential implementation barriers by designing curriculum with these challenges in mind. That is, the curriculum was designed as a flexible set of modules that target specific sub-skills, with each module consisting of pre-lab (hypothetical) and in-lab (real) activities. Each module was designed around a curricular framework such that an adopting institution can use the materials as written, or can incorporate their existing equipment and experiments into the framework with minimal effort. Other non-traditional approaches have also been experimented with, such as the work by Sobhanzadeh, Kalman, and Thompson ( 2017 ), which targets typical misconceptions by using conceptual questions to engage students in making a prediction, designing and conducting a related experiment, and determining whether or not the results support the hypothesis.
Another challenge for inquiry labs is the assessment of skills-based learning outcomes. For assessment of scientific reasoning, a new instrument on inquiry in scientific thinking analytics and reasoning (iSTAR) has been developed, which can be easily implemented across large numbers of students as both a pre- and post-test to assess gains. iSTAR assesses reasoning skills necessary in the systematical conduct of scientific inquiry, which includes the ability to explore a problem, formulate and test hypotheses, manipulate and isolate variables, and observe and evaluate the consequences (see www.istarassessment.org ). The new instrument expands upon the commonly used classroom test of scientific reasoning (Lawson, 1978 , 2000 ), which has been identified with a number of validity weaknesses and a ceiling effect for college students (Bao, Xiao, Koenig, & Han, 2018 ).
Many education innovations need supporting infrastructures that can ensure adoption and lasting impact. However, making large-scale changes to current education settings can be risky, if not impossible. New education approaches, therefore, need to be designed to adapt to current environmental constraints. Since higher-end skills are a primary focus of twenty-first Century learning, which are most effectively developed in inquiry-based group settings, transforming current lecture and lab courses into this new format is critical. Although this transformation presents great challenges, promising solutions have already emerged from various research efforts. Perhaps the biggest challenge is for STEM educators and researchers to form an alliance to work together to re-engineer many details of the current education infrastructure in order to overcome the multitude of implementation obstacles.
This paper attempts to identify a few central ideas to provide a broad picture for future research and development in physics education, or STEM education in general, to promote twenty-first Century learning. Through a synthesis of the existing literature within the authors’ limited scope, a number of views surface.
Education is a service to prepare (not to select) the future workforce and should be designed as learner-centered, with the education goals and teaching-learning methods tailored to the needs and characteristics of the learners themselves. Given space constraints, the reader is referred to the meta-analysis conducted by Freeman et al. ( 2014 ), which provides strong support for learner-centered instruction. The changing world of the twenty-first Century informs the establishment of new education goals, which should be used to guide research and development of teaching and learning for present day students. Aligned to twenty-first Century learning, the new science standards have set the goals for STEM education to transition towards promoting deep learning of disciplinary knowledge, thereby building upon decades of research in PER, while fostering a wide range of general high-end cognitive and non-cognitive abilities that are transferable across all disciplines.
Following these education goals, more research is needed to operationally define and assess the desired high-end reasoning abilities. Building on a clear definition with effective assessments, a large number of empirical studies are needed to investigate how high-end abilities can be developed in parallel with deep learning of concepts, such that what is learned can be generalized to impact the development of curriculum and teaching methods which promote skills-based learning across all STEM fields. Specifically for PER, future research should emphasize knowledge integration to promote deep conceptual understanding in physics along with inquiry learning to foster scientific reasoning. Integration of physics learning in contexts that connect to other STEM disciplines is also an area for more research. Cross-cutting, interdisciplinary connections are becoming important features of the future generation physics curriculum and defines how physics should be taught collaboratively with other STEM courses.
This paper proposed meaningful areas for future research that are aligned with clearly defined education goals for twenty-first Century learning. Based on the existing literature, a number of challenges are noted for future directions of research, including the need for:
clear and operational definitions of goals to guide research and practice
concrete operational definitions of high-end abilities for which students are expected to develop
effective assessment methods and instruments to measure high-end abilities and other components of twenty-first Century learning
a knowledge base of the curriculum and teaching and learning environments that effectively support the development of advanced skills
integration of knowledge and ability development regarding within-discipline and cross-discipline learning in STEM
effective means to disseminate successful education practices
The list is by no means exhaustive, but these themes emerge above others. In addition, the high-end abilities discussed in this paper focus primarily on scientific reasoning, which is highly connected to other skills, such as critical thinking, systems thinking, multivariable modeling, computational thinking, design thinking, etc. These abilities are expected to develop in STEM learning, although some may be emphasized more within certain disciplines than others. Due to the limited scope of this paper, not all of these abilities were discussed in detail but should be considered an integral part of STEM learning.
Finally, a metacognitive position on education research is worth reflection. One important understanding is that the fundamental learning mechanism hasn’t changed, although the context in which learning occurs has evolved rapidly as a manifestation of the fast-forwarding technology world. Since learning is a process at the interface between a learner’s mind and the environment, the main focus of educators should always be on the learner’s interaction with the environment, not just the environment. In recent education developments, many new learning platforms have emerged at an exponential rate, such as the massive open online courses (MOOCs), STEM creative labs, and other online learning resources, to name a few. As attractive as these may be, it is risky to indiscriminately follow trends in education technology and commercially-incentivized initiatives before such interventions are shown to be effective by research. Trends come and go but educators foster students who have only a limited time to experience education. Therefore, delivering effective education is a high-stakes task and needs to be carefully and ethically planned and implemented. When game-changing opportunities emerge, one needs to not only consider the winners (and what they can win), but also the impact on all that is involved.
Based on a century of education research, consensus has settled on a fundamental mechanism of teaching and learning, which suggests that knowledge is developed within a learner through constructive processes and that team-based guided scientific inquiry is an effective method for promoting deep learning of content knowledge as well as developing high-end cognitive abilities, such as scientific reasoning. Emerging technology and methods should serve to facilitate (not to replace) such learning by providing more effective education settings and conveniently accessible resources. This is an important relationship that should survive many generations of technological and societal changes in the future to come. From a physicist’s point of view, a fundamental relation like this can be considered the “mechanics” of teaching and learning. Therefore, educators and researchers should hold on to these few fundamental principles without being distracted by the surfacing ripples of the world’s motion forward.
Availability of data and materials
Not applicable.
Abbreviations
American Association of Physics Teachers
Investigative Science Learning Environment
Inquiry in Scientific Thinking Analytics and Reasoning
Massive open online course
New Generation Science Standards
- Physics education research
Science Technology Engineering and Math
Alonso, M. (1992). Problem solving vs. conceptual understanding. American Journal of Physics , 60 (9), 777–778. https://doi.org/10.1119/1.17056 .
Article Google Scholar
Anderson, L. W., Krathwohl, D. R., Airasian, P. W., Cruikshank, K. A., Mayer, R. E., Pintrich, P. R., … Wittrock, M. C. (2001). A taxonomy for learning, teaching, and assessing: A revision of Bloom’s taxonomy of educational objectives, abridged edition . White Plains: Longman.
Ates, S., & Cataloglu, E. (2007). The effects of students’ reasoning abilities on conceptual understandings and problem-solving abilities in introductory mechanics. European Journal of Physics , 28 , 1161–1171.
Bagno, E., Eylon, B.-S., & Ganiel, U. (2000). From fragmented knowledge to a knowledge structure: Linking the domains of mechanics and electromagnetism. American Journal of Physics , 68 (S1), S16–S26.
Bailin, S. (1996). Critical thinking. In J. J. Chambliss (Ed.), Philosophy of education: An encyclopedia , (vol. 1671, pp. 119–123). Routledge.
Bangert-Drowns, R. L., & Bankert, E. (1990). Meta-analysis of effects of explicit instruction for critical thinking. Research report. ERIC Number: ED328614.
Google Scholar
Bao, L., Cai, T., Koenig, K., Fang, K., Han, J., Wang, J., … Wu, N. (2009). Learning and scientific reasoning. Science , 323 , 586–587. https://doi.org/10.1126/science.1167740 .
Bao, L., & Redish, E. F. (2001). Concentration analysis: A quantitative assessment of student states. American Journal of Physics , 69 (S1), S45–S53.
Bao, L., & Redish, E. F. (2006). Model analysis: Representing and assessing the dynamics of student learning. Physical Review Special Topics-Physics Education Research , 2 (1), 010103.
Bao, L., Xiao, Y., Koenig, K., & Han, J. (2018). Validity evaluation of the Lawson classroom test of scientific reasoning. Physical Review Physics Education Research , 14 (2), 020106.
Beichner, R. J., Saul, J. M., Abbott, D. S., Morse, J. J., Deardorff, D., Allain, R. J., … Risley, J. S. (2007). The student-centered activities for large enrollment undergraduate programs (SCALE-UP) project. Research-Based Reform of University Physics , 1 (1), 2–39.
Binkley, M., Erstad, O., Herman, J., Raizen, S., Ripley, M., & Rumble, M. (2010). Draft White paper defining 21st century skills . Melbourne: ACTS.
Bloom, B. S., Engelhart, M. D., Furst, E. J., Hill, W. H., & Krathwohl, D. R. (1956). Taxonomy of educational objectives: Handbook 1: Cognitive domain . New York: Longman.
Bransford, J. D., Brown, A. L., & Cocking, R. R. (2000). How people learn , (vol. 11). Washington, DC: National Academy Press.
Brown, A. (1989). Analogical learning and transfer: What develops? In S. Vosniadu, & A. Ortony (Eds.), Similarity and analogical reasoning , (pp. 369–412). New York: Cambridge U.P.
Chapter Google Scholar
Cavallo, A. M. L., Rozman, M., Blickenstaff, J., & Walker, N. (2003). Learning, reasoning, motivation, and epistemological beliefs: Differing approaches in college science courses. Journal of College Science Teaching , 33 (3), 18–22.
Chen, Z., & Klahr, D. (1999). All other things being equal: Acquisition and transfer of the control of variables strategy. Child Development , 70 , 1098–1120.
Chi, M. T., Bassok, M., Lewis, M. W., Reimann, P., & Glaser, R. (1989). Self-explanations: How students study and use examples in learning to solve problems. Cognitive Science , 13 (2), 145–182.
Chi, M. T., Feltovich, P. J., & Glaser, R. (1981). Categorization and representation of physics problems by experts and novices. Cognitive Science , 5 (2), 121–152.
Chi, M. T., & Slotta, J. D. (1993). The ontological coherence of intuitive physics. Cognition and Instruction , 10 (2–3), 249–260.
Chi, M. T., Slotta, J. D., & De Leeuw, N. (1994). From things to processes: A theory of conceptual change for learning science concepts. Learning and Instruction , 4 (1), 27–43.
Chi, M. T. H. (1992). Conceptual change within and across ontological categories: Examples from learning and discovery in science. In R. N. Giere (Ed.), Cognitive models of science . Minneapolis: University of Minnesota Press.
Chiu, M. H. (2001). Algorithmic problem solving and conceptual understanding of chemistry by students at a local high school in Taiwan. Proceedings-National Science Council Republic of China Part D Mathematics Science and Technology Education , 11 (1), 20–38.
Chiu, M.-H., Guo, C. J., & Treagust, D. F. (2007). Assessing students’ conceptual understanding in science: An introduction about a national project in Taiwan. International Journal of Science Education , 29 (4), 379–390.
Clement, J. (1982). Students’ preconceptions in introductory mechanics. American Journal of Physics , 50 (1), 66–71.
Coletta, V. P., & Phillips, J. A. (2005). Interpreting FCI scores: Normalized gain, preinstruction scores, and scientific reasoning ability. American Journal of Physics , 73 (12), 1172–1182.
Cracolice, M. S., Deming, J. C., & Ehlert, B. (2008). Concept learning versus problem solving: A cognitive difference. Journal of Chemical Education , 85 (6), 873.
De Jong, T., & Ferguson-Hesler, M. G. M. (1986). Cognitive structure of good and poor problem solvers in physics. Journal of Educational Psychology , 78 , 279–288.
DiSessa, A. A. (1993). Toward an epistemology of physics. Cognition and Instruction , 10 (2–3), 105–225.
Duit, R., & Treagust, D. F. (2003). Conceptual change: A powerful framework for improving science teaching and learning. International Journal of Science Education , 25 (6), 671–688.
Dykstra Jr., D. I., Boyle, C. F., & Monarch, I. A. (1992). Studying conceptual change in learning physics. Science Education , 76 (6), 615–652.
Ennis, R. (1993). Critical thinking assessment. Theory Into Practice , 32 (3), 179–186.
Etkina, E., & Van Heuvelen, A. (2001). Investigative science learning environment: Using the processes of science and cognitive strategies to learn physics. In Proceedings of the 2001 physics education research conference , (pp. 17–21). Rochester.
Etkina, E., Van Heuvelen, A., White-Brahmia, S., Brookes, D. T., Gentile, M., Murthy, S., … Warren, A. (2006). Scientific abilities and their assessment. Physical Review Special Topics-Physics Education Research , 2 (2), 020103.
Eylon, B.-S., & Reif, F. (1984). Effects of knowledge organization on task performance. Cognition and Instruction , 1 (1), 5–44.
Fabby, C., & Koenig, K. (2013). Relationship of scientific reasoning to solving different physics problem types. In Proceedings of the 2013 Physics Education Research Conference, Portland, OR .
Facione, P. A. (1990). Critical thinking: A statement of expert consensus for purposes of educational assessment and instruction – The Delphi report . Millbrae: California Academic Press.
Ferguson-Hesler, M. G. M., & De Jong, T. (1990). Studying physics texts: Differences in study processes between good and poor solvers. Cognition and Instruction , 7 (1), 41–54.
Fisher, A. (2001). Critical thinking: An introduction . Cambridge: Cambridge University Press.
Freeman, S., Eddy, S. L., McDonough, M., Smith, M. K., Okoroafor, N., Jordt, H., & Wenderoth, M. P. (2014). Active learning increases student performance in science, engineering, and mathematics. Proceedings of the National Academy of Sciences , 111 (23), 8410–8415.
Glaser, E. M. (1941). An experiment in the development of critical thinking . New York: Teachers College, Columbia University.
Hake, R. R. (1992). Socratic pedagogy in the introductory physics laboratory. The Physics Teacher , 30 , 546.
Hake, R. R. (1998). Interactive-engagement versus traditional methods: A six-thousand-student survey of mechanics test data for introductory physics courses. American Journal of Physics , 66 (1), 64–74.
Halloun, I. A., & Hestenes, D. (1985a). The initial knowledge state of college physics students. American Journal of Physics , 53 (11), 1043–1055.
Halloun, I. A., & Hestenes, D. (1985b). Common sense concepts about motion. American Journal of Physics , 53 (11), 1056–1065.
Halpern, D. F. (1999). Teaching for critical thinking: Helping college students develop the skills and dispositions of a critical thinker. New Directions for Teaching and Learning , 80 , 69–74. https://doi.org/10.1002/tl.8005 .
Hardiman, P. T., Dufresne, R., & Mestre, J. P. (1989). The relation between problem categorization and problem solving among experts and novices. Memory & Cognition , 17 (5), 627–638.
Heller, J. I., & Reif, F. (1984). Prescribing effective human problem-solving processes: Problem description in physics. Cognition and Instruction , 1 (2), 177–216.
Hoellwarth, C., Moelter, M. J., & Knight, R. D. (2005). A direct comparison of conceptual learning and problem solving ability in traditional and studio style classrooms. American Journal of Physics , 73 (5), 459–462.
Hofstein, A., & Lunetta, V. N. (1982). The role of the laboratory in science teaching: Neglected aspects of research. Review of Educational Research , 52 (2), 201–217.
Hsu, L., Brewe, E., Foster, T. M., & Harper, K. A. (2004). Resource letter RPS-1: Research in problem solving. American Journal of Physics , 72 (9), 1147–1156.
Jensen, J. L., & Lawson, A. (2011). Effects of collaborative group composition and inquiry instruction on reasoning gains and achievement in undergraduate biology. CBE - Life Sciences Education , 10 , 64–73.
Johnson, M. A., & Lawson, A. E. (1998). What are the relative effects of reasoning ability and prior knowledge on biology achievement in expository and inquiry classes? Journal of Research in Science Teaching , 35 (1), 89–103.
Kalman, C., & Lattery, M. (2018). Three active learning strategies to address mixed student epistemologies and promote conceptual change. Frontiers in ICT , 5 (19), 1–9.
Karplus, R. (1964). The science curriculum improvement study. Journal of College Science Teaching , 2 (4), 293–303.
Kim, E., & Pak, S.-J. (2002). Students do not overcome conceptual difficulties after solving 1000 traditional problems. American Journal of Physics , 70 (7), 759–765.
Koenig, K., Schen, M., & Bao, L. (2012). Explicitly targeting pre-service teacher scientific reasoning abilities and understanding of nature of science through an introductory science course. Science Educator , 21 (2), 1–9.
Koenig, K., Schen, M., Edwards, M., & Bao, L. (2012). Addressing STEM retention through a scientific thought and methods course. Journal of College Science Teaching , 41 , 23–29.
Koenig, K., Wood, K., Bortner, L., & Bao, L. (2019). Modifying traditional labs to target scientific reasoning. Journal of College Science Teaching , 48 (5), 28-35.
Kozminski, J., Beverly, N., Deardorff, D., Dietz, R., Eblen-Zayas, M., Hobbs, R., … Zwickl, B. (2014). AAPT recommendations for the undergraduate physics laboratory curriculum , (pp. 1–29). American Association of Physics Teachers Retrieved from https://www.aapt.org/Resources/upload/LabGuidlinesDocument_EBendorsed_nov10.pdf .
Kuhn, D. (1992). Thinking as argument. Harvard Educational Review , 62 (2), 155–178.
Larkin, J., McDermott, J., Simon, D. P., & Simon, H. A. (1980). Expert and novice performance in solving physics problems. Science , 208 (4450), 1335–1342.
Laws, P. W. (2004). Workshop physics activity guide, module 4: Electricity and magnetism. In Workshop physics activity guide . Wiley-VCH.
Lawson, A. E. (1978), The development and validation of a classroom test of formal reasoning, Journal of Research in Science Teaching , 15 (1), 11–24.
Lawson, A. E. (1992). The development of reasoning among college biology students - a review of research. Journal of College Science Teaching , 21 , 338–344.
Lawson, A. E. (2000). Classroom test of scientific reasoning: Multiple choice version, based on Lawson, A. E. 1978. Development and validation of the classroom test of formal reasoning. Journal of Research in Science Teaching , 15 (1), 11–24.
Lee, H. S., Liu, O. L., & Linn, M. C. (2011). Validating measurement of knowledge integration in science using multiple-choice and explanation items. Applied Measurement in Education , 24 (2), 115–136.
Linn, M. C. (2005). The knowledge integration perspective on learning and instruction. In R. K. Sawyer (Ed.), The Cambridge handbook of the learning sciences , (pp. 243–264). Cambridge: Cambridge University Press. https://doi.org/10.1017/CBO9780511816833.016 .
Lipman, M. (2003). Thinking in education , (2nd ed., ). Cambridge: Cambridge University Press.
Liu, X. (2010). Science and engineering education sources. Using and developing measurement instruments in science education: A Rasch modeling approach . Charlotte: IAP Information Age Publishing.
Marzano, R. J., Brandt, R. S., Hughes, C. S., Jones, B. F., Presseisen, B. Z., Rankin, S. C., et al. (1988). Dimensions of thinking, a framework for curriculum and instruction . Alexandria: Association for Supervision and Curriculum Development.
Mazur, E. (1997). Peer instruction: A user’s manual . Upper Saddle River: Prentice Hall.
McDermott, L. C. (1996). Physics by Inquiry: An Introduction to the Physical Sciences . John Wiley & Sons, New York, NY.
McDermott, L. C., & Redish, E. F. (1999). Resource letter: PER-1: Physics education research. American Journal of Physics , 67 (9), 755–767.
McKeachie, W. J. (1986). Teaching and learning in the college classroom: A review of the research literature . Ann Arbor: National Center for Research to Improve Postsecondary Teaching and Learning.
Meltzer, D. E., & Otero, V. K. (2015). A brief history of physics education in the United States. American Journal of Physics , 83 (5), 447–458.
Meltzer, D. E., & Thornton, R. K. (2012). Resource letter ALIP-1: Active-learning instruction in physics. American Journal of Physics , 80 (6), 478–496.
Minstrell, J. (1992). Facets of students’ knowledge and relevant instruction. In R. Duit, F. Goldberg, & H. Niedderer (Eds.), Proceedings of the international workshop: Research in physics learning- theoretical issues and empirical studies , (pp. 110–128). The Institute for Science Education.
Nakhleh, M. B. (1993). Are our students conceptual thinkers or algorithmic problem solvers? Identifying conceptual students in general chemistry. Journal of Chemical Education , 70 (1), 52. https://doi.org/10.1021/ed070p52 .
Nakhleh, M. B., & Mitchell, R. C. (1993). Concept learning versus problem solving: There is a difference. Journal of Chemical Education , 70 (3), 190. https://doi.org/10.1021/ed070p190 .
National Research Council (2011). Assessing 21st century skills: Summary of a workshop . Washington, DC: The National Academies Press. https://doi.org/10.17226/13215 .
Book Google Scholar
National Research Council (2012a). Education for life and work: Developing transferable knowledge and skills in the 21st century . Washington, DC: The National Academies Press.
National Research Council (2012b). A framework for K-12 science education: Practices, crosscutting concepts, and core ideas . Washington, DC: National Academies Press.
National Research Council (2012c). Discipline-based education research: Understanding and improving learning in undergraduate science and engineering . Washington, DC: National Academies Press.
National Science & Technology Council (2018). Charting a course for success: America’s strategy for STEM education . Washington, DC: Office of Science and Technology Policy.
NCET. (1987). Critical thinking as defined by the National Council for excellence in critical thinking, statement by Michael Scriven & Richard Paul, presented at the 8th annual conference on critical thinking and education reform. Retrieved December 4, 2018, from http://www.criticalthinking.org/pages/defining-critical-thinking/766 .
Nordine, J., Krajcik, J., & Fortus, D. (2011). Transforming energy instruction in middle school to support integrated understanding and future learning. Science Education , 95 (4), 670–699.
Nurrenbern, S. C., & Pickering, M. (1987). Concept learning versus problem solving: Is there a difference? Journal of Chemical Education , 64 (6), 508.
Paul, R. (1990). Critical thinking: What every person needs to survive in a rapidly changing world . Rohnert Park: Center for Critical Thinking and Moral Critique.
Perkins, D. N., & Salomon, G. (1989). Are cognitive skills context-bound? Educational Researcher , 18 (1), 16–25.
Posner, G., Strike, K., Hewson, P., & Gertzog, W. (1982). Accommodation of a scientific conception: Toward a theory of conceptual change. Science Education , 66 (2), 211–227.
Reay, N. W., Bao, L., Li, P., Warnakulasooriya, R., & Baugh, G. (2005). Toward the effective use of voting machines in physics lectures. American Journal of Physics , 73 (6), 554–558.
Reimers, F. M., & Chung, C. K. (Eds.) (2016). Teaching and learning for the twenty-first century: Educational goals, policies and curricula from six nations . Cambridge: Harvard Education Press.
Salomon, G., & Perkins, D. N. (1989). Rocky roads to transfer: Rethinking mechanism of a neglected phenomenon. Educational Psychologist , 24 (2), 113–142.
Schoenfeld, A. H., & Herrmann, D. J. (1982). Problem perception and knowledge structure in expert and novice mathematical problem solvers. Journal of Experimental Psychology: Learning, Memory, and Cognition , 8 (5), 484.
Shaw, V. F. (1996). The cognitive processes in informal reasoning. Thinking and Reasoning , 2 (1), 51–80.
She, H., & Liao, Y. (2010). Bridging scientific reasoning and conceptual change through adaptive web-based learning. Journal of Research in Science Teaching , 47 (1), 91–119.
Shen, J., Liu, O. L., & Chang, H.-Y. (2017). Assessing students’ deep conceptual understanding in physical sciences: An example on sinking and floating. International Journal of Science and Mathematics Education , 15 (1), 57–70. https://doi.org/10.1007/s10763-015-9680-z .
Siegel, H. (1988). Educating reason: Rationality, critical thinking and education , (vol. 1). New York: Routledge.
Slotta, J. D., Chi, M. T., & Joram, E. (1995). Assessing students’ misclassifications of physics concepts: An ontological basis for conceptual change. Cognition and Instruction , 13 (3), 373–400.
Smith III, J. P., DiSessa, A. A., & Roschelle, J. (1994). Misconceptions reconceived: A constructivist analysis of knowledge in transition. The Journal of the Learning Sciences , 3 (2), 115–163.
Smith, M. U. (1992). Expertise and organization of knowledge: Unexpected differences among genetic counselors, faculty members and students on problem categorization tasks. Journal of Research in Science Teaching , 29 (2), 179–205.
Sobhanzadeh, M., Kalman, C. S., & Thompson, R. I. (2017). Labatorials in introductory physics courses. European Journal of Physics , 38 , 1–18.
Sokoloff, D. R., Thornton, R. K., & Laws, P. W. (2011). RealTime physics: Active learning laboratories . New York: Wiley.
Stamovlasis, D., Tsaparlis, G., Kamilatos, C., Papaoikonomou, D., & Zarotiadou, E. (2005). Conceptual understanding versus algorithmic problem solving: Further evidence from a national chemistry examination. Chemistry Education Research and Practice , 6 (2), 104–118.
Tanenbaum, C. (2016). STEM 2026: A vision for innovation in STEM education . Washington, DC: US Department of Education.
Thornton, R. K., & Sokoloff, D. R. (1998). Assessing student learning of Newton’s laws: The force and motion conceptual evaluation and the evaluation of active learning laboratory and lecture curricula. American Journal of Physics , 66 (4), 338–352.
Trowbridge, L. W., Bybee, R. W., & Powell, J. C. (2000). Teaching secondary school science: Strategies for developing scientific literacy . Upper Saddle River: Merrill-Prentice Hall.
United States Chamber of Commerce (2017). Bridging the soft skills gap: How the business and education sectors are partnering to prepare students for the 21 st century workforce . Washington DC: Center for Education and Workforce, U.S. Chamber of Commerce Foundation.
Veldhuis, G. H. (1990). The use of cluster analysis in categorization of physics problems. Science Education , 74 (1), 105–118.
Vosniadou, S., Vamvakoussi, X., & Skopeliti, I. (2008). The framework theory approach to the problem of conceptual change. In S. Vosniadou (Ed.), International handbook of research on conceptual change . New York: Routledge.
Wexler, P. (1982). Structure, text, and subject: A critical sociology of school knowledge. In M. W. Apple (Ed.), Cultural and economic reproduction in education: Essays on class, ideology and the state . London: Routledge & Regan Paul.
Zeineddin, A., & Abd-El-Khalick, F. (2010). Scientific reasoning and epistemological commitments: Coordination of theory and evidence among college science students. Journal of Research in Science Teaching , 47 (9), 1064–1093.
Zimmerman, C. (2000). The development of scientific reasoning skills. Developmental Review , 20 (1), 99–149.
Download references
Acknowledgements
The research is supported in part by NSF Awards DUE-1431908 and DUE-1712238. Any opinions, findings, and conclusions or recommendations expressed in this paper are those of the author(s) and do not necessarily reflect the views of the National Science Foundation.
The research is supported in part by NSF Awards DUE-1431908 and DUE-1712238.
Author information
Authors and affiliations.
The Ohio State University, Columbus, OH, 43210, USA
University of Cincinnati, Cincinnati, OH, 45221, USA
Kathleen Koenig
You can also search for this author in PubMed Google Scholar
Contributions
LB developed the concept, wrote a significant portion of the review and position, and synthesized the paper. KK wrote and edited a significant portion of the paper. Both authors read and approved the final manuscript.
Corresponding author
Correspondence to Lei Bao .
Ethics declarations
Competing interests.
The authors declare that they have no competing interests.
Additional information
Publisher’s note.
Springer Nature remains neutral with regard to jurisdictional claims in published maps and institutional affiliations.
Rights and permissions
Open Access This article is distributed under the terms of the Creative Commons Attribution 4.0 International License ( http://creativecommons.org/licenses/by/4.0/ ), which permits unrestricted use, distribution, and reproduction in any medium, provided you give appropriate credit to the original author(s) and the source, provide a link to the Creative Commons license, and indicate if changes were made.
Reprints and permissions
About this article
Cite this article.
Bao, L., Koenig, K. Physics education research for 21 st century learning. Discip Interdscip Sci Educ Res 1 , 2 (2019). https://doi.org/10.1186/s43031-019-0007-8
Download citation
Received : 17 April 2019
Accepted : 13 June 2019
Published : 28 November 2019
DOI : https://doi.org/10.1186/s43031-019-0007-8
Share this article
Anyone you share the following link with will be able to read this content:
Sorry, a shareable link is not currently available for this article.
Provided by the Springer Nature SharedIt content-sharing initiative
- Twenty-first century learning
- STEM education
- Scientific reasoning
- Deep learning

- Physics Magazine
- Physical Review Journals
- Physics Today
- Other APS Publications
- March Meeting
- April Meeting
- Meeting Calendar
- Abstract Submission
- Meeting Archive
- Policies & Guidelines
- International Affairs
- Public Engagement
- Women in Physics
- Minorities in Physics
- LGBT Physicists
- Industrial Physics
- Renew Membership
- Member Directory
- My Member Profile
- Member Services
- APS Chapters
- Action Center
- Reports & Studies
- APS Statements
- Contact APS Government Affairs
- Physics Jobs
- Becoming a Physicist
- Career Guidance
- Tools for Career Advisors
- Statistical Data
- News & Announcements
- Press Releases
- Social Media
- Mission, Vision, Values
- Strategic Plan
- Society Governance
- Society History
- Donate to APS
- Become a Member
July/August 2021 (Volume 30, Number 7)
The aps topical group on physics education research.
By Abigail Dove
The APS Topical Group on Physics Education Research (GPER) is a home for members interested in research on the learning and teaching of physics—a broad, multidisciplinary area encompassing everything from institutional practices to neural and cognitive processes to the social and contextual components of physics educational practices.
Physics education research (PER) falls within the purview of the emerging area of discipline-based education research, a field that focuses on the comprehensive study of learning and teaching in particular academic disciplines, most often (but not exclusively) at the university level.
Researchers in the GPER community are also focused on wider aspects of the teaching, learning, and practice of physics that extend beyond physics content itself. “For example, we need to ensure that students' attitudes and habits of mind in physics classes are strengthened by our courses,” explained GPER chair-elect Mila Kryjevskaia (North Dakota State University). “We also need to ensure that our instructional efforts support all students rather than a subpopulation of students. Do we provide adequate support for those students who need it most? Do our instructional activities and techniques help build classroom environments that promote diversity and inclusion, as well as enjoyment and appreciation of science? How can we support faculty who would like to implement research-based instructional approaches in their classrooms? High school teachers are critical for shaping the future of the physics community, what do we need to do to prepare and support them better?”
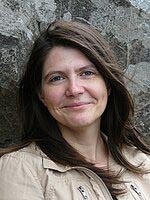
Mila Kryjevskaia
While PER represents a relatively young sub-field of physics, it is one of the most well-established areas of discipline-based education research, more mature than corresponding education research in other STEM fields like biology, chemistry, and engineering. GPER was established in 2014 with the goal of integrating the rapidly expanding PER field into the broader physics community. Since then, the field has continued to thrive and expand, both in size and scope. “It is not uncommon anymore to see a physics education researcher on a faculty roster in a department of physics,” according to Kryjevskaia. “Often, PER faculty contribute substantially to the health of the department by bringing in knowledge in education to the department, as well as contributing to generalized knowledge about teaching and learning physics.”
Mirroring the growth of the PER field, GPER is a fast-growing unit, with membership consistently above 600. Notably, almost half of GPER’s members are students or early career scientists, signifying the vibrance of this new field and foreshadowing continued growth of the PER community. At more than 30%, GPER also boasts one of the highest proportions of female membership across all APS units.
GPER has a strong presence at the annual APS April Meeting, sponsoring or co-sponsoring at least two sessions every year, often in collaboration with the Forum on Education (FEd; see APS News June 2020 ). “APS meetings are a great opportunity for me to engage in conversations with other APS members who do not specialize in physics education research,” noted Kryjevskaia. “When a GPER-sponsored session is full of faces I do not recognize, I consider it to be a great success.” After all, even physicists who are not directly involved in undergraduate teaching have a stake in physics education, whether that takes the form of mentoring graduate students and post-docs or training new hires in industry.
In the age of coronavirus, many APS members may be thinking especially deeply about physics education—particularly the urgent issue of how to optimize pedagogy for remote learning. To this end, several of this year’s GPER-sponsored sessions focused on pandemic-related topics, including recent research on impact of remote learning in the physics classroom, strategies for teaching physics online, and perspectives on how the graduate admissions process might be re-thought in the post-COVID world.
For APS members interested in learning more about physics education research in general, the Physical Review Physics Education Research (PRPER) journal published by APS is an excellent resource. The most recent edition of PRPER highlights issues ranging from student experimentation decisions in introductory laboratories to the effects of transforming homework assignments using the principles of deliberate practice and to the effect of culture on women physicists’ career choices .
Additionally, many findings from GPER researchers are synthesized in the Effective Practices for Physics Programs (EP3) Project , an initiative led by APS in collaboration with the American Association of Physics Teachers (AAPT) to document evidence-based recommendations and best practices for undergraduate physics education. The aim of EP3 is to develop a resource that will help a wide variety of physics programs strive for excellence within their particular opportunities and constraints, recognizing that what makes for a thriving physics program at a private university is not necessarily the same as that for a liberal arts college, a large state school, or a community college.
Going forward, the GPER executive committee’s goals for the unit are two-fold: Looking inward, to continue the advancement of PER as a sub-discipline of physics; looking outward, to advocate for further integration of PER into the broader physics community. “We need to sustain and develop venues that foster new conversations between researchers in physics education and APS members who do not specialize in PER,” explained Kryjevskaia. “I would like GPER to continue promoting an environment that welcomes and supports everyone, particularly those who are just beginning a new journey and who rely on our community for support, regardless of whether that support is needed in the areas of research, teaching, mentoring, or career development.”
Overall, GPER stands out a vibrant community for sharing ideas, resources, and research about physics education. More information on this unit can be found on the GPER website.
The author is a freelance writer in Stockholm, Sweden.
©1995 - 2024, AMERICAN PHYSICAL SOCIETY APS encourages the redistribution of the materials included in this newspaper provided that attribution to the source is noted and the materials are not truncated or changed.
Editor: David Voss Staff Science Writer: Leah Poffenberger Contributing Correspondents: Sophia Chen, Alaina G. Levine
APS News Home
Issue Table of Contents
APS News Archives
Contact APS News Editor

Become an APS Member Submit a Meeting Abstract Submit a Manuscript Find a Journal Article Donate to APS
Renew Membership Join an APS Unit Update Contact Information
Information for
Librarians Authors Referees Media Students
The American Physical Society (APS) is a nonprofit membership organization working to advance the knowledge of physics.
© 2024 American Physical Society | Privacy Policy | Contact Us 1 Physics Ellipse, College Park, MD 20740-3844 | (301) 209-3200
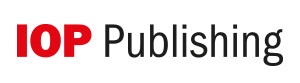
We apologize for the inconvenience...
To ensure we keep this website safe, please can you confirm you are a human by ticking the box below.
If you are unable to complete the above request please contact us using the below link, providing a screenshot of your experience.
https://ioppublishing.org/contacts/
Supporting physics teaching with research-based resources
- Expert Recommendations
Welcome to PhysPort, the go-to place for physics faculty to find resources based on physics education research (PER) to support your teaching. Learn more...
- find a new teaching method
- find curricular materials
- train my LAs or TAs
- run a faculty workshop
- find an assessment
- analyze assessment results
- assess advanced physics content or skills
Troubleshooting
- engaging students
- arguments for skeptical colleagues
- something else
Featured Expert Recommendations
Where can i find good questions to use with clickers or peer instruction.
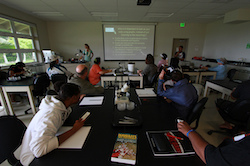
Peer Instruction , CAE Think-Pair-Share , Technology-Enhanced Formative Assessment , clickers
What makes research-based teaching methods in physics work?
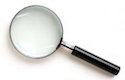
active learning
Best practices for whiteboarding in the physics classroom
whiteboarding , best practices , SCALE-UP , Modeling Instruction
View all Expert Recommendations »

Latest news from PhysPort
Get a sneak preview of our new designs for PhysPort , coming in 2024.
Free open-source research-based curricula: PhysPort now hosts collections of curricula.
Use our Periscope collection of video lessons in your online LA/TA training class: Contact us for more details.
Find a physics education consultant: Find external evaluators, researchers, writers, editors, and more to help with your project.
Curated collection of free wave and optics resources for your online class: Simulations & models, virtual labs, data analysis tools, video collections, and free during COVID-19.
PhysPort Data Explorer
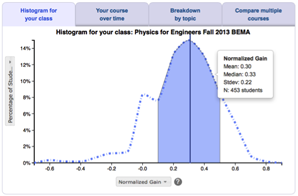
Home | Expert Recommendations | Teaching Methods | Assessment | Workshops | Data Explorer | About | Help | Contact | Terms | Privacy | My Account
CERN Accelerating science
Physics Education Research
The CERN Convention cites engaging the public with fundamental research as a vital part of the Organization's mission. Indeed, CERN and the experimental collaborations it hosts have a considerable history of high-quality communication and outreach efforts that reach a wide range of international audiences. These activities are complemented by prominent educational programmes, such as CERN's residential programmes for high-school science teachers and students . In 2009, CERN's Physics Education Research (PER) activities were launched to support and further develop educational activities and to provide consultancy for all actors in the Organization's education and outreach efforts.
Today, CERN's PER team comprises an international team of staff scientists, postdoctoral research fellows, doctoral students, and visiting master students who perform education research in the field of particle physics. Among the various research activities are quantitative and qualitative studies focusing on teachers' professional development and students' conceptions and interest, as well as the iterative development of low-cost, hands-on experimental equipment for the classroom and the education labs in CERN's Science Gateway . For a detailed overview of all ongoing and completed research projects and to meet CERN's PER team, have a look here!
Latest Publications
- Kersting, M., Blair, D., Sandrelli, S., Sherson, J. & Woithe, J. ( 2023 ). Making an IMPRESSion: mapping out future directions in modern physics education. Physics Education, 59(1), 015501, article
- Dahlkemper, M. N., Lahme, S. Z. & Klein, P. ( 2023 ). How do physics students evaluate artificial intelligence responses on comprehension questions? A study on the perceived scientific accuracy and linguistic quality of ChatGPT. Physical Review Physics Education Research, 19(1), 010142, article
- Woithe, J., Boselli, M., Chatzidaki, P., Dahlkemper, M. N., Duggan, R., Durey, G., Herff, N., Kranjc Horvat, A., Molaro, D., Scheerer, G. W., Schmeling, S., Thill, P. T., Wiener, J. & Zoechling, S. ( 2022 ). Higgs in a box: investigating the nature of a scientific discovery. The Physics Educator, 4(4), 1-15, article
- Dahlkemper, M.N., Klein, P., Müller, A., Schmeling, S.M. & Wiener, J. ( 2022 ). Opportunities and Challenges of Using Feynman Diagrams with Upper Secondary Students. Physics, 4(4), 1331-1347, article
- Kranjc Horvat, A., Wiener, J., Schmeling, S. & Borowski, A. ( 2022 ). What Does the Curriculum Say? Review of the Particle Physics Content in 27 High-School Physics Curricula. Physics, 4(4), 1278-1298, article
- Zöchling, S., Hopf, M., Woithe, J. & Schmeling, S. ( 2022 ). Students’ interest in particle physics: Conceptualisation, instrument development, and evaluation using Rasch theory and analysis, International Journal of Science Education, article
- Chatzidaki, P., Woithe, J., David, A. & Dunford, M. ( 2022 ). Ten things we’ve learned about the Higgs boson in the past ten years, Science in School, 59, article
- Kranjc Horvat, A., Boselli, M., Chatzidaki, P., Dahlkemper, M. N., Duggan, R., Durey, G., Herff, N., Molaro, D., Scheerer, G. W., Schmeling, S., Thill, P. T., Wiener, J., Woithe, J. & Zöchling, S. ( 2022 ). The mystery box challenge: explore the nature of science, Science in School, 59, article
- Woithe, J., Müller, A., Schmeling, S. & Kuhn, J. ( 2022 ). Motivational outcomes of the science outreach lab S'Cool LAB at CERN: A multilevel analysis. Journal of Research in Science Teaching, article
- Zöchling, S., Hopf, M., Woithe, J. & Schmeling, S. ( 2022 ). MAKE IT MATTER: How to foster interest in particle physics by setting it in meaningful contexts. PoS (EPS-HEP2021), 889, 1-4, proceedings
- Kranjc Horvat, A., Wiener, J., Schmeling, S. & Borowski, A. ( 2022 ). Learning Goals of Professional Development Programs at Science Research Institutions: A Delphi Study with Different Stakeholder Groups. Journal of Science Teacher Education, 33(1), 32-54, article
For a complete overview of all publications of CERN's PER team, have a look here!
Acting as an international PER hub, CERN actively collaborates with many partners throughout CERN's Member States and beyond on a variety of projects, many of which are related to doctoral students' research projects. If your institute or organisation is not yet in the non-exhaustive list below, feel free to get in touch - we should talk!

- Return to: AIP Conference Proceedings
- Return to: AIP Publishing Books
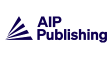
The International Handbook of Physics Education Research: Teaching Physics
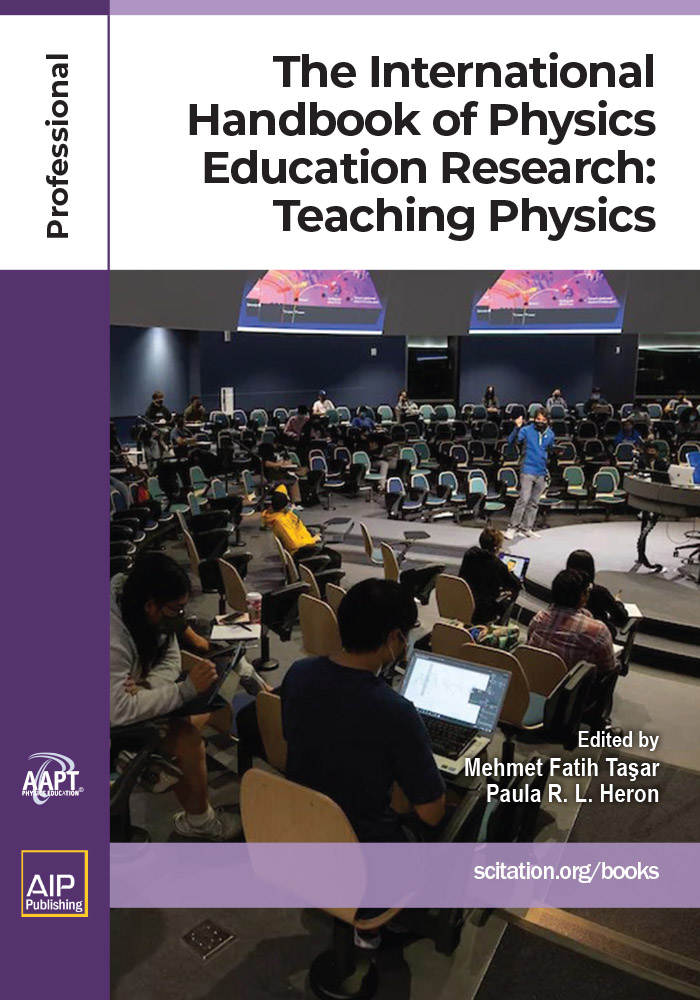
By Mehmet Fatih Taşar and Paula R. L. Heron

Title information
The International Handbook of Physics Education Research: Teaching Physics covers the topics of educational technologies, learning environments, teacher education, and assessment in relation to physics education. As the field of physics education research grows, it is increasingly difficult for newcomers to gain an appreciation of the major findings across all sub-domains, discern global themes, and recognize gaps in the literature. The current volume incorporates the understanding of both physics and education concepts and provides an extensive review the literature in a wide range of important topics.
The International Handbook of Physics Education Research: Teaching Physics includes:
- Various teaching perspectives including active learning, the modeling method, and teaching environments.
- Distance/online education, formative and summative assessment, and assessment in online education.
- Gender, race, and ethnicity equity.
Readers will find this comprehensive treatment of the literature useful in understanding physics education research and extending to all the physical sciences including chemistry, mathematics, astronomy, and other related disciplines.
Secondary menu
- Privacy Policy
- Terms & Conditions
Copyright 2017 AIP Publishing LLC. All rights reserved. Powered by Sheridan Press
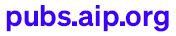
Department of Physics & Astronomy
- Brief History of the Department
- Department Buildings
- Department Openings
- Artist in Residence Program
- Image Gallery
- Travel and Business
- Equity and Inclusion
- Departmental Code of Conduct
- Appointment Stream Faculty
- Tenured/Tenure Stream
- Part-Time Faculty
- Graduate Students
- Undergraduate Students
- Astrophysics/Cosmology Faculty
- Biological Physics Faculty
- Condensed Matter Faculty
- Particle Physics Faculty
- Physics Education Research Faculty
- How to Apply
- M.S. Program
- PhD Program
- Teaching Assistants
- Financial Assistance
- Graduate Student Life
- Graduation Procedures
- Graduate Applicants FAQ
- Why Study Physics?
- Degree Programs
- Fellowships & Awards
- Research Opportunities
- Scholarship & Intern Opportunities
- Society of Physics Students
- Support Physics & Astronomy
- Update My Information
- Distinguished Alumni Awards
- Alumni Directory
- Alumni Spotlight
- Alumni Lectures
- Event Archive
- Prospective Students
Physics Education Research
Physics Education Research// Melanie Good
Physics Education Research focuses on improving the teaching of physics by understanding the cognitive mechanisms which either aid or hinder student learning, and developing pedagogical techniques to improve learning. Chandralekha Singh has led numerous studies documenting student difficulties in both introductory and advanced physics classes, and cognitive strategies for improving problem solving ability. The Singh research group has built and evaluated research-based tutorials for use in both introductory and advanced physics courses.
The group also investigates innovations that can benefit the Department's and the University's teaching mission. Some examples are the establishment of the Physics Exploration Center, where students in introductory courses can experiment independently; the installation of student response systems (or "clickers") in all four of the department's larger lecture halls, which enable the instructor to monitor student responses to questions posed during classes; and the construction of an introductory physics classroom designed to facilitate studio-style interactive teaching.
Chandralekha Singh directs the Discipline-Based Science Education Research Center (dB-SERC) , which aims to improve science teaching in the Dietrich School of Arts and Sciences by helping faculty developand implement courses which use research-based pedagogical techniques.The Center hosts meetings of faculty members from different departments focusing on various aspects of science pedagogy, brings speakers to campus to lead workshops about the teaching and learning of science, and offers support for specific efforts to enhance the curriculum in particular courses.
Russell Clark has studied ways to increase the effectiveness of undergraduate student laboratories, including lab experiments oriented towards health sciences students and peer review of lab reports. Matteo Broccio has been working on a transformation of the algebra-based introductory courses to a more student-centered instruction with increased focus on the physiological relevance of Physics principles. From a research perspective, he is investigating the impact of self-diagnostic instruments on performance, attitude and belief systems of students in large enrollment classes. David Nero seeks to use technology to improve physics education in large-enrollment classes. His most recent work has been in the creation of online lectures and supporting material for the introductory calculus-based physics sequence.
- Physics and Science Education Research

The Wieman group’s research falls into the following main categories:
Uses of PhET Simulations across the K-16 curriculum PhET simulations (phet.colorado.edu) are interactive science simulations that are extensively used by a wide range of students and teachers (around 100 million uses this year in a variety of science classrooms, from elementary schools through college). Because simulations are used by such a wide range of students, we are researching what and how students of different ages and scientific backgrounds learn from them. How effective are PhET simulations at motivating and engaging such a wide range of students in science? We aim to determine which aspects of the simulations best support learning across a range of student ages and backgrounds, and what sorts of supporting materials or instruction are most effective for those different students. In addition, we are looking into what learning outcomes PhET simulations facilitate, from learning specific science concepts, to exploring the underlying mechanisms of the phenomenon, to developing inquiry or metacognitive skills.
Identifying student inquiry skills We are examining the interplay between content knowledge and problem solving strategies, and how this relation is mediated by technology and socioemotional factors. Problem solving strategies are too often studied separately from social and emotional contexts and are evaluated too universally regardless of the accompanying content knowledge. The goals of this research are, primarily, to identify which problem solving strategies pave the way to expertise and which strategies paralyze the experts; and, secondarily, how socioemotional factors and technology use can positively or negatively contribute to these processes.
Cognitive principles for instructional design Although current “active learning” efforts have been shown to provide better learning outcomes than traditional instructional methods, there is currently little guidance on how to design such materials to best support learning. We are designing, implementing, and studying instructional materials that take into account findings on human cognition, such as the benefits of inventing from a series of contrasting cases (e.g. Schwartz et al., 2011). By studying the efficacy of these materials, we hope to provide instructors, curriculum developers, and researchers with new principles for designing effective instructional materials for typical classroom instruction.
STEM Expertise--measuring, teaching, and learning We look at the characteristics that distinguish expert thinking across multiple science and engineering and medical disciplines. We are developing better ways to measure the degree to which students at various levels have developed these thinking skills, and in laboratory and classroom environments we test various ways to improve the acquisition of these skills. This work is heavily guided by research in cognitive psychology.
Related Faculty

Carl Wieman
Physics Education Research
Physics Education Research (PER) works toward theoretical understanding and practical advancement of instruction, specifically concerned with the disciplinary knowledge and practices of physics. Researchers work within and contribute to the PER community , situated mainly in departments of physics, as well as the (much larger) communities of the learning sciences and education research .
At Tufts, PER takes place in collaborations between the Department of Physics and Astronomy and the Department of Education , and with researchers at other universities and research organizations. Our research ranges from foundational theory to developing curriculum and instructional practices, focused mainly at college levels but spanning levels from elementary school through graduate.
Research Faculty
David Hammer 's group studies the dynamics of how students approach learning , in particular episodes and developing over time, and responsive teaching —instruction that attends to and engages with the substance of students' thinking.
Roger Tobin works on curriculum and teacher professional development for elementary school science, as well as on implementing and disseminating research-based instructional approaches at Tufts.
Tim Atherton studies project-based approaches to learning, particularly around integration of computation into the physics curriculum.
home - login - register
- FFPER: Puget Sound 2024
- FFPER Series
- Gordon Series
- PERC Series
- All Conferences
PER Programs
- Community Events
- Community Announcements
- For the Media
- PERTG & PERLOC
- PER SOLO Group
- PER Graduate Students
- Course Packages
- Student Activities
Research Instruments
- Pedagogy Guides
- Suggest a Material
- Advanced Search
- Suggest an Article
- Suggest a Dissertation
- Reviews in PER
- PERC Proceedings
Education research is our focus
Welcome to PER-Central, a resource collection for physics education researchers. Here you may find articles, theses and dissertations, research groups, curricular material, and news and events of interest to the PER Community. Learn more about PER-Central .
PER Literature
Search the literature by:
Or find an article by topic:
- Basic Research
- Achievement
- Alternative Conceptions
- Communication
- Learning Theory
- Problem Solving
- Research Design & Methodology
- Sample Population
- Societal Issues
- Student Characteristics
- Teacher Characteristics
- Applied Research
- Active Learning
- Classroom Management
- Curriculum Development
- Informal Education
- Instructional Material Design
- Learning Environment
- Professional Development
- Recruitment
- School Improvement
- Teacher Preparation
By Topic: Astronomy , Classical Mechanics , Electricity & Magnetism , Fluid Mechanics , General Physics , Mathematical Tools , Modern Physics , Optics , Oscillations & Waves , Other Sciences , Quantum Physics , Relativity , Thermo & Stat Mech
Volume 2 of Reviews in PER is dedicated to helping researchers get started in Physics Education Research. In this review article, a member of the PER community presents an overview of an area of PER:
Getting Started in Qualitative Physics Education Research by V. K. Otero and D. B. Harlow .
Find more articles introducing PER research methods in Volume 2 of Reviews in PER .
Community Resources
- PER-Central Forums

Browse the different PER groups and their descriptions on the PER Program Map .
For the Classroom

PhysPort The PhysPort helps physics educators apply PER results, assessments, and the teaching methods based on these results.
Find more PER-based Curricular Materials: Course Packages | Student Activities | Pedagogy Guides

Attitudes and Approaches to Problem Solving Survey The AAPS, developed by Mason and Singh, is designed to evaluate students' attitudes and approaches towards physics problem solving. The survey is based on investigations of responses from introductory physics students, graduate students, and faculty members. It expands upon the Attitudes towards Problem Solving survey (Marx and Cummings, 2007) to consider approaches to problem solving and different levels of problem solving expertise.
Find more assessment instruments
FFPER: Puget Sound 2024 The fifth FFPERPS (Foundations and Frontiers of Physics Education Research: Puget Sound) conference will be held June 20-23, 2024 at the North Cascades Environmental Learning Center.
Physics Education Research Conference 2024 PERC 2024 will be held July 10-11, 2024 in Boston, Massachusetts. The theme will be "Bridging the Institutional Gap: PER at Primarily Undergraduate Four Year Institution, Two-Year College, and K-12 Levels."
More events
Latest Additions


Physics Education Research
Research and Outreach
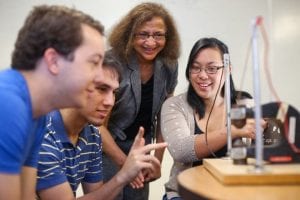
Physics Education Research at UCF is led by two tenure-track assistant professors. Both Dr. Jackie Chini and Dr. Zhongzhou Chen received their Ph.D.s in physics for research in physics education. We study issues relevant to the evolving landscape of higher education in physics and collaborate with colleagues in related disciplines.
Dr. Jackie Chini’s group examines how to adapt active learning strategies for diverse student populations and institutional contexts. She has received funding through the NSF-WIDER program to study diverse implementations of SCALE-UP across the country and through the NSF-IUSE program to study the efficacy of popular active learning strategies for students with executive function disorders, common among students with ADHD, learning disabilities, autism spectrum disorder and student veterans.
Dr. Zhongzhou Chen is interested in combining online education technology with learning science to shape the future of STEM education. Collaborating with the Center for Distributed Learning at UCF (online.ucf.edu), he designs and develops online learning modules based on the concept of deliberate practice. He is also interested in measuring and modeling th e fundamental cognitive process behind physics problem solving.
Many other faculty, including both tenure-line faculty and lecturers, work on physics education research projects and have interest in improving physics education.
- Dr. Ahlam Al-Rawi formed the Physics Education Research by Lecturers (PERL) group at UCF. Lecturers at UCF teach many of our large courses, so they are able to collect data from large samples. The members of PERL have PhDs in physics for research in a variety of disciplines, which enables them to connect with a wide array of student interests and difficulties. Dr. Al-Rawi is also interested in implementing and creating curriculum for student-centered learning and leads the department’s Outreach Committee and Student Life Committees. Her interests lie in using interdisciplinary thinking to attract more students to science and in supporting integration of all students in departmental research opportunities.
- Dr. Jeffrey Bindell was one of the leaders of the revival of SCALE-UP/studio-mode instruction in introductory physics at UCF. He is interested in assessing student learning across very different instructional strategies, such as comparing lecture-based and project-based introductory courses.
- Dr. Enrique Del Barco was an iSTEM Faculty Fellow in 2015-2016. He has recently led the creation of a service-learning nanoscience minor, a physics summer camp (PHySICOS), and a Physical Science capstone research program for undergraduates. His educational activities focus on increasing enrollment, reducing attrition and facilitating transition into the profession in STEM fields.
- Dr. Archana Dubey was part of the team that reinvigorated SCALE-UP/studio physics at UCF. She is interested in improving student learning in introductory and modern physics. She has presented talks and posters about scaffolding students’ problem solving with multi-part problems in Physics II.
- Costas Efthimiou was a co-PI on UCF’s ICUBED (Innovations through Institutional Integration) NSF-funded project.
- Dr. Elena Flitsiyan is interested in incorporating service-learning in the physics curriculum and using technology to enhance learning. In first semester calculus-based physics, her students completed the service-learning project “Physics of the Car Accident: Building a Safe Campus by Solving Physics Problems”.
- Dr. Talat Rahman was awarded an American Physical Society (APS) Physics Teacher Education Coalition (PhysTEC) grant to improve physics teacher recruitment and education.
- Dr. Christos Velisarris is a member of PERL. His general education science course “Physics in Films” uses scenes from movies to motivate students to learn about physical science
Physical Review Physics Education Research
- Collections
- Editorial Team
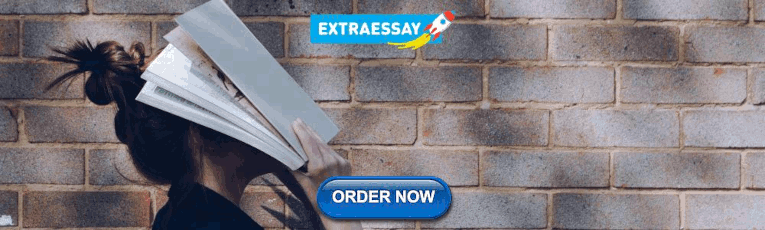
Volume 18, Issue 1
January - june 2022, editorials and announcements, editorial: research on advancing equity is critical for physics, charles henderson and michael thoennessen, phys. rev. phys. educ. res. 18 , 010001 (2022) – published 11 april 2022, highlighted articles, featured in physics editors' suggestion, lgbt + physicists: harassment, persistence, and uneven support, ramón s. barthelemy, madison swirtz, savannah garmon, elizabeth h. simmons, kyle reeves, michael l. falk, wouter deconinck, elena a. long, and timothy j. atherton, phys. rev. phys. educ. res. 18 , 010124 (2022) – published 28 march 2022, viewpoint: making physics inclusive to lgbtq+ folks.
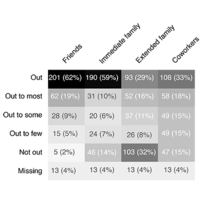
LGBT+ members of the physics community who have negative perceptions of workplace climate and who observe exclusionary behavior are more likely to consider leaving the discipline.
Show Abstract
Featured in physics, not feeling recognized as a physics person by instructors and teaching assistants is correlated with female students’ lower grades, sonja cwik and chandralekha singh, phys. rev. phys. educ. res. 18 , 010138 (2022) – published 31 may 2022, : keeping women in physics is more than a numbers game.
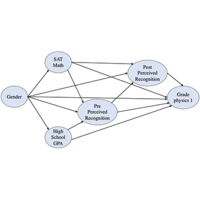
Women in introductory physics are less likely than men to think that others perceive them as being a physics person.
Students’ sense of belonging in introductory physics course for bioscience majors predicts their grade
Phys. rev. phys. educ. res. 18 , 010139 (2022) – published 31 may 2022.
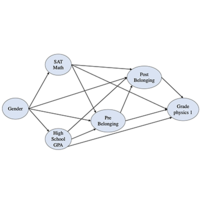
In introductory physics courses where men outnumber women, mens’ sense of belonging increases while womens’ does not.
Editors' Suggestion
Impact of virtual research experience for undergraduates experiences on students’ psychosocial gains during the covid-19 pandemic, dina zohrabi alaee, micah k. campbell, and benjamin m. zwickl, phys. rev. phys. educ. res. 18 , 010101 (2022) – published 4 january 2022.
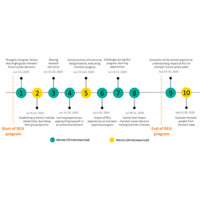
Remote research experience for undergraduates programs should be considered as a means to expand access to research experiences for students.
Evaluating the role of student preference in physics lab group equity
N. g. holmes, grace heath, katelynn hubenig, sophia jeon, z. yasemin kalender, emily stump, and eleanor c. sayre, phys. rev. phys. educ. res. 18 , 010106 (2022) – published 20 january 2022.
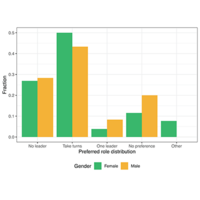
Inequities in student lab group experiences are due to structural aspects of the learning environment; instructors must institute policies and practices to push against gender norms and stereotypes.
Cross-disciplinary learning index: A quantitative measure of cross-disciplinary learning about energy
Todd haskell, emily borda, and andrew boudreaux, phys. rev. phys. educ. res. 18 , 010108 (2022) – published 24 january 2022.
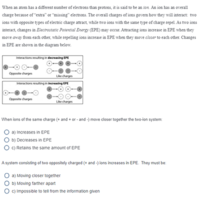
The development and validation of techniques to measure cross-disciplinary learning: an example in the context of energy.
Increased learning in a college physics course with timely use of short multimedia summaries
Spencer dunleavy, greg kestin, kristina callaghan, logan mccarty, and louis deslauriers, phys. rev. phys. educ. res. 18 , 010110 (2022) – published 28 january 2022.
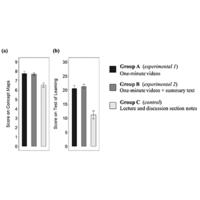
Carefully designed one-minute videos and short text summaries following a lecture can significantly increase students’ understanding of the material and their ability to organize the material into useful mental frameworks.
Skills-focused lab instruction improves critical thinking skills and experimentation views for all students
Cole walsh, h. j. lewandowski, and n. g. holmes, phys. rev. phys. educ. res. 18 , 010128 (2022) – published 11 april 2022.
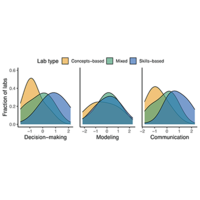
Labs focused on skills improve critical thinking skills and experimentation views for all students compared with labs focused on reinforcing lecture concepts.
Identifying students’ mental models of the apparent motion of the Sun and stars
Hans bekaert, hans van winckel, wim van dooren, an steegen, and mieke de cock, phys. rev. phys. educ. res. 18 , 010130 (2022) – published 20 april 2022.
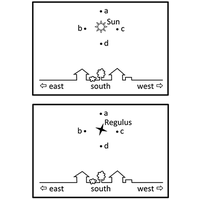
There are five different mental models that students use to reason about the apparent motion of the Sun and stars.
Examining the effects of lab instruction and gender composition on intergroup interaction networks in introductory physics labs
Meagan sundstrom, david g. wu, cole walsh, ashley b. heim, and n. g. holmes, phys. rev. phys. educ. res. 18 , 010102 (2022) – published 4 january 2022.
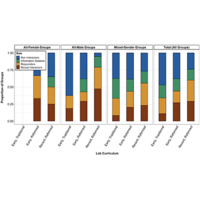
In introductory physics labs, direct prompts from the lab instructor to interact with other groups leads to more and longer interactions.
Development and validation of the Conceptual Survey on Wave Optics
Karolina matejak cvenic, maja planinic, ana susac, lana ivanjek, katarina jelicic, and martin hopf, phys. rev. phys. educ. res. 18 , 010103 (2022) – published 10 january 2022.
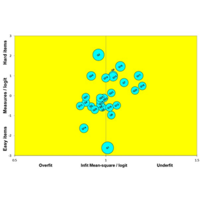
The conceptual survey on wave optics is a valid and reliable 26-item multiple-choice instrument suitable for use as a post-test for high school students.
Investigating unprompted and prompted diagrams generated by physics majors during problem solving
Michael vignal and bethany r. wilcox, phys. rev. phys. educ. res. 18 , 010104 (2022) – published 12 january 2022.
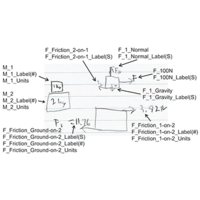
On some types of problems many students can select the correct answer without drawing a diagram; on other types of problems many student who drew diagrams were unable to select the correct answer.
Working together or alone, near, or far: Social connections and communities of practice in in-person and remote physics laboratories
Drew j. rosen and angela m. kelly, phys. rev. phys. educ. res. 18 , 010105 (2022) – published 12 january 2022.
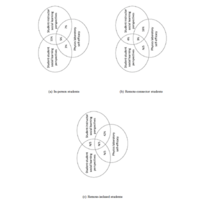
Students in remote undergraduate physics labs form weak communities of practice with one another, and their instructors exist outside of this community structure.
Examining the relationship between informal science experiences and physics identity: Unrealized possibilities
Zahra hazari, remy dou, gerhard sonnert, and philip m. sadler, phys. rev. phys. educ. res. 18 , 010107 (2022) – published 20 january 2022.
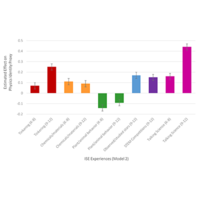
Informal learning experiences in physics reinforce stereotypical practices, fail to intersect in positive ways with the biological sciences, and continue to be less accessible to students from historically marginalized groups.
Assessing the impact of metacognitive postreflection exercises on problem-solving skillfulness
Aaron reinhard, alex felleson, paula c. turner, and maxwell green, phys. rev. phys. educ. res. 18 , 010109 (2022) – published 24 january 2022.
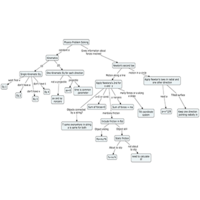
The efficacy of metacognitive reflections on recently completed work to improve retention of newly learned problem-solving techniques may be highly context dependent.
Defining and assessing understandings of evidence with the assessment rubric for physics inquiry: Towards integration of argumentation and inquiry
C. f. j. pols, p. j. j. m. dekkers, and m. j. de vries, phys. rev. phys. educ. res. 18 , 010111 (2022) – published 15 february 2022.
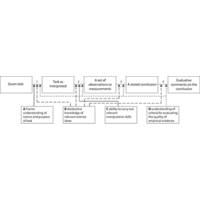
An approach to assessing the presence and application of inquiry knowledge required for engaging in physics based on an observation of actions and decisions.
Improving test security and efficiency of computerized adaptive testing for the Force Concept Inventory
Jun-ichiro yasuda, michael m. hull, and naohiro mae, phys. rev. phys. educ. res. 18 , 010112 (2022) – published 17 february 2022.
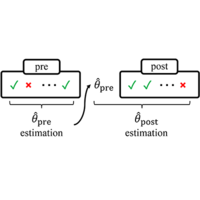
The accuracy and precision of the full-length Force Concept Inventory can be achieved with fewer items via computerized adaptive testing.
Characterizing active learning environments in physics using latent profile analysis
Kelley commeford, eric brewe, and adrienne traxler, phys. rev. phys. educ. res. 18 , 010113 (2022) – published 23 february 2022.
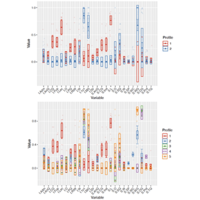
Characterizing active learning environments at fine grained level allows for an understanding of the distinguishing characteristics of pedagogies in physics.
Participation, performance, and outcomes in an undergraduate physics degree: Perspectives on gender and socioeconomic factors
Rachel e. harrington, job h. j. thijssen, and judy hardy, phys. rev. phys. educ. res. 18 , 010114 (2022) – published 23 february 2022.
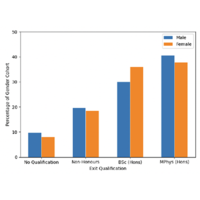
Institutional contexts are different and to support equitable practices and outcomes each institution collects and make decisions based on appropriate data about the academic performance and experience of their students.
Theoretical model and quantitative assessment of scientific thinking and reasoning
Lei bao, kathleen koenig, yang xiao, joseph fritchman, shaona zhou, and cheng chen, phys. rev. phys. educ. res. 18 , 010115 (2022) – published 23 february 2022.
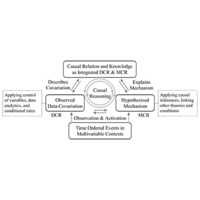
A new instrument to assess scientific reasoning built from a modeling framework that integrates research in scientific and causal reasoning.
Framework for unpacking students’ mindsets in physics by gender
Z. yasemin kalender, emily marshman, christian d. schunn, timothy j. nokes-malach, and chandralekha singh, phys. rev. phys. educ. res. 18 , 010116 (2022) – published 28 february 2022.
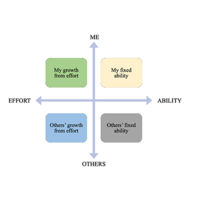
Female physics students are more likely than male physics students to believe that innate talent is needed to excel in physics, and that they may not have that talent.
Development and validation of an astronomy self-efficacy instrument for understanding and doing
Rachel freed, david mckinnon, michael fitzgerald, and christina m. norris, phys. rev. phys. educ. res. 18 , 010117 (2022) – published 28 february 2022.
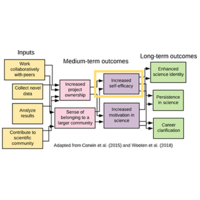
It is important to development domain-specific self-efficacy instruments as a way to assess and improve instruction with an example from astronomy.
Observing whiteness in introductory physics: A case study
Amy d. robertson and w. tali hairston, phys. rev. phys. educ. res. 18 , 010119 (2022) – published 11 march 2022.
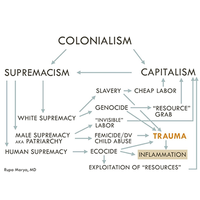
Whiteness shapes many practices in physics classrooms that are often thought to be neutral.
Impact of introductory physics for the life sciences in a senior biology capstone course
Benjamin d. geller, jack rubien, sara m. hiebert, and catherine h. crouch, phys. rev. phys. educ. res. 18 , 010120 (2022) – published 11 march 2022.
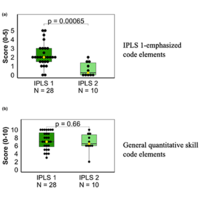
Biology majors who take the introductory physics for life sciences course are more likely to reason quantitatively and mechanistically about diffusive phenomena and to successfully coordinate between multiple representations of diffusive processes.
Instructor interactions in traditional and nontraditional labs
David g. wu, ashley b. heim, meagan sundstrom, cole walsh, and n. g. holmes, phys. rev. phys. educ. res. 18 , 010121 (2022) – published 14 march 2022.
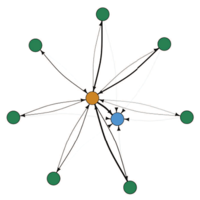
The amount of interaction between instructors and students during lab is more a product of the instructional design than an individual instructor’s implementation of that design.
Designing and implementing materials on quantum computing for secondary school students: The case of teleportation
Sara satanassi, elisa ercolessi, and olivia levrini, phys. rev. phys. educ. res. 18 , 010122 (2022) – published 14 march 2022.
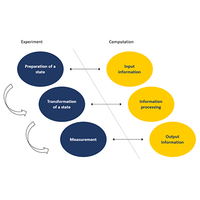
Designing an inclusive and productive learning environment for the teaching of quantum computing in secondary schools.
History of science based dialogues on sound waves: From sound atoms to phonons
Alexander volfson, haim eshach, and yuval ben-abu, phys. rev. phys. educ. res. 18 , 010123 (2022) – published 18 march 2022.
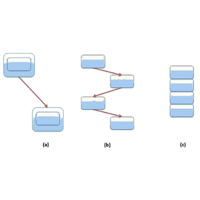
An instructional technique that makes use of whole class discussions based on the history of science.
What decisions do experts make when doing back-of-the-envelope calculations?
Gabriel murillo-gonzalez and eric w. burkholder, phys. rev. phys. educ. res. 18 , 010125 (2022) – published 5 april 2022.
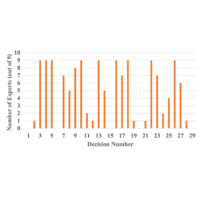
Solving back-of-the-envelope problems does not require different skills than solving other kinds of problems that physicists encounter in their research.
Measuring the level of homework answer copying during COVID-19 induced remote instruction
Zhongzhou chen, phys. rev. phys. educ. res. 18 , 010126 (2022) – published 5 april 2022.
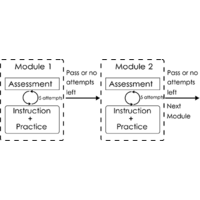
Most students do not engage in answer copying behaviors to complete online homework assignments in introductory calculus-based physics.
Learning entropy among peers through the lens of coordination class theory
Juan velasco, laura buteler, carlos briozzo, and enrique coleoni, phys. rev. phys. educ. res. 18 , 010127 (2022) – published 7 april 2022.
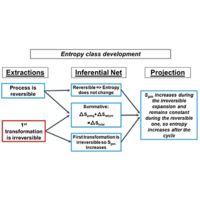
Using coordination class theory to observe learning at both the individual and the group level.
Impacts on student learning, confidence, and affect in a remote, large-enrollment, course-based undergraduate research experience in physics
Alexandra werth, colin g. west, and h. j. lewandowski, phys. rev. phys. educ. res. 18 , 010129 (2022) – published 13 april 2022.
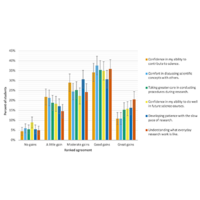
Implementation and assessment of a large-scale, introductory, online physics course-based undergraduate research experience.
Assessing the impact of introductory physics for the life sciences on students’ ability to build complex models
Benjamin d. geller, maya tipton, brandon daniel-morales, nikhil tignor, calvin white, and catherine h. crouch, phys. rev. phys. educ. res. 18 , 010131 (2022) – published 20 april 2022.
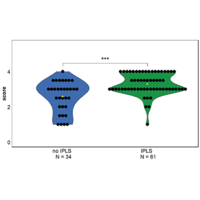
Introductory physics for the life sciences students are more successfully than students in a typical physics course at combining multiple ideas to solve problems in new contexts.
Evaluation of high school student responses to the Colorado Learning Attitudes about Science Survey
Julian s. martins and william e. lindsay, phys. rev. phys. educ. res. 18 , 010132 (2022) – published 25 april 2022.
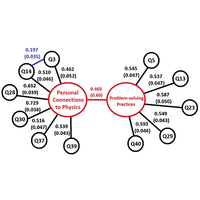
Developing an attitudes and beliefs survey structure for K-12 physics courses aligned with the Next Generation Science Standards.
Motivations for using the item response theory nominal response model to rank responses to multiple-choice items
Trevor i. smith and nasrine bendjilali, phys. rev. phys. educ. res. 18 , 010133 (2022) – published 25 april 2022.
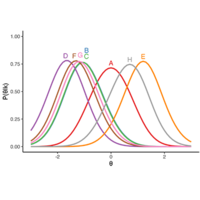
New insights on multiple-choice assessments based on similarities between the item response theory nominal response model and the probability function of the canonical ensemble with degenerate energy states.
Detecting preservice teachers’ visual attention under prediction and nonprediction conditions with eye-tracking technology
Qiuye li, shaorui xu, yilin chen, chuting lu, and shaona zhou, phys. rev. phys. educ. res. 18 , 010134 (2022) – published 27 april 2022.
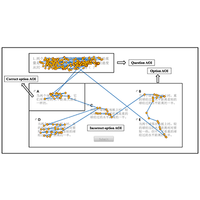
In an eye-tracking study, preservice teachers who correctly predicted student responses paid attention to different areas than those teachers who did not correctly predict student responses.
Investigating students’ views of experimental physics in German laboratory classes
E. teichmann, h. j. lewandowski, and m. alemani, phys. rev. phys. educ. res. 18 , 010135 (2022) – published 27 april 2022.
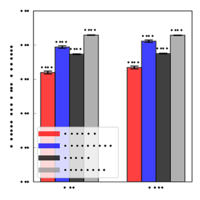
A German language version of the E-CLASS instrument is available for understanding students views of experimental physics.
Problem solving in basic physics: Effective self-explanations based on four elements with support from retrieval practice
Vegard gjerde, vegard havre paulsen, bodil holst, and stein dankert kolstø, phys. rev. phys. educ. res. 18 , 010136 (2022) – published 2 may 2022.
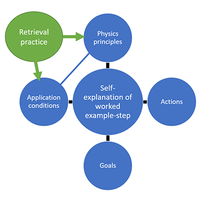
Retrieval practice of physics principles and their conditions of application before self-explanation can have a positive effect on posttest problem-solving scores and can increase the quality of students’ self-explanations.
High school students’ perceptions on the relevance of inquiry-oriented instructional labs as introduction to an extended research project
David perl-nussbaum and edit yerushalmi, phys. rev. phys. educ. res. 18 , 010137 (2022) – published 25 may 2022.
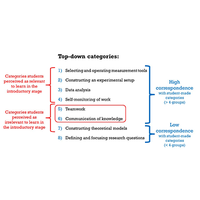
Investigating high school student perceptions of the value of different inquiry practices in a learning progression and interpreting these finding using the lens of boundary crossing.
Difficulties in understanding mechanical waves: Remediated by problem-based instruction
Stella teddy kanyesigye, jean uwamahoro, and imelda kemeza, phys. rev. phys. educ. res. 18 , 010140 (2022) – published 2 june 2022.
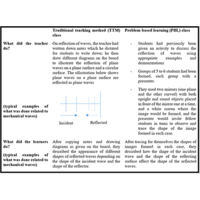
Implementation of problem based learning in secondary schools in Western Uganda improves student understanding of mechanical waves.
Classification of open-ended responses to a research-based assessment using natural language processing
Joseph wilson, benjamin pollard, john m. aiken, marcos d. caballero, and h. j. lewandowski, phys. rev. phys. educ. res. 18 , 010141 (2022) – published 2 june 2022.
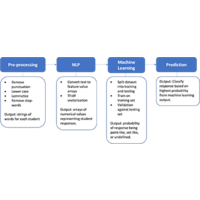
A natural language processing model can be trained to categorize open-ended responses to the physics measurement questionnaire as well as a pair of human coders.
Do female and male students’ physics motivational beliefs change in a two-semester introductory physics course sequence?
Yangqiuting li and chandralekha singh, phys. rev. phys. educ. res. 18 , 010142 (2022) – published 3 june 2022.
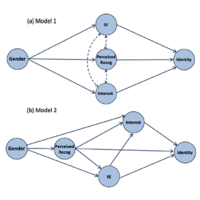
The current learning environment in traditionally taught introductory physics courses does not improve students’ physics motivational beliefs, and initial gender differences in these beliefs becomes larger.
Mediating role of personality in the relation of gender to self-efficacy in physics and mathematics
Rachel henderson, dona hewagallage, jake follmer, lynnette michaluk, jessica deshler, edgar fuller, and john stewart, phys. rev. phys. educ. res. 18 , 010143 (2022) – published 3 june 2022.
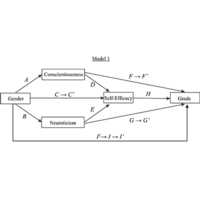
A substantial amount of the often-reported differences in self-efficacy between men and women may result from gender differences in the tendency to experience anxiety and the tendency to conscientiously complete tasks.
Exploring student ideas on change of basis in quantum mechanics
Giaco corsiglia, benjamin p. schermerhorn, homeyra sadaghiani, armando villaseñor, steven pollock, and gina passante, phys. rev. phys. educ. res. 18 , 010144 (2022) – published 6 june 2022.
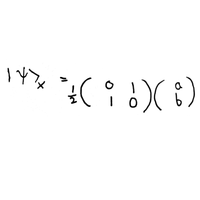
Quantum mechanics students grapple with basis and change of basis in a variety of ways.
From Cartesian coordinates to Hilbert space: Supporting student understanding of basis in quantum mechanics
Benjamin p. schermerhorn, giaco corsiglia, homeyra sadaghiani, gina passante, and steven pollock, phys. rev. phys. educ. res. 18 , 010145 (2022) – published 8 june 2022.
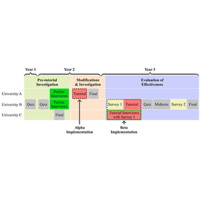
The iteratively designed tutorial effectively helps students make sense of and carry out a change of basis in quantum mechanics by drawing an analogy between quantum state vectors and 2D Cartesian vectors.
Long-term collaboration with strong friendship ties improves academic performance in remote and hybrid teaching modalities in high school physics
Javier pulgar, diego ramírez, abigail umanzor, cristian candia, and iván sánchez, phys. rev. phys. educ. res. 18 , 010146 (2022) – published 13 june 2022.
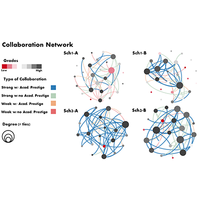
Strong peer-to-peer collaborations in a physics class require time to develop and can result in higher grades.
Workplace climate for LGBT + physicists: A view from students and professional physicists
Ramón s. barthelemy, bryce e. hughes, madison swirtz, matthew mikota, and timothy j. atherton, phys. rev. phys. educ. res. 18 , 010147 (2022) – published 13 june 2022.
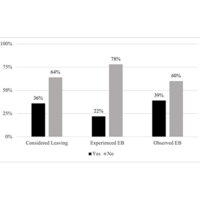
Actively cultivating an LGBT-inclusive work environment may have a more profound impact on retaining LGBT physicists than only removing exclusive behaviors and practices.
Harnessing active engagement in educational videos: Enhanced visuals and embedded questions
Greg kestin and kelly miller, phys. rev. phys. educ. res. 18 , 010148 (2022) – published 21 june 2022.
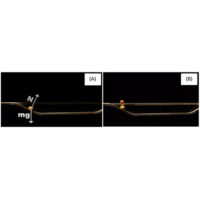
Instructonal physics videos are most effective when they include enhanced visuals and embedded questions.
Examining the relation of high school preparation and college achievement to conceptual understanding
Dona hewagallage, elaine christman, and john stewart, phys. rev. phys. educ. res. 18 , 010149 (2022) – published 28 june 2022.
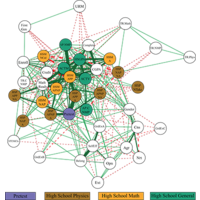
Gender differences observed in pretest and post-test scores were changed little by controlling for either high school preparation or noncognitive factors.
Today’s interdisciplinary quantum information classroom: Themes from a survey of quantum information science instructors
Josephine c. meyer, gina passante, steven j. pollock, and bethany r. wilcox, phys. rev. phys. educ. res. 18 , 010150 (2022) – published 30 june 2022.
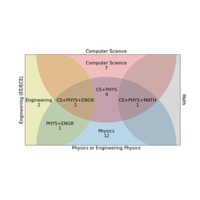
There is not a canonical approach to introduction to quantum information science courses.
REVIEW ARTICLES
Critical race and feminist standpoint theories in physics education research: a historical review and potential applications, miguel rodriguez, ramón barthelemy, and melinda mccormick, phys. rev. phys. educ. res. 18 , 013101 (2022) – published 25 february 2022.
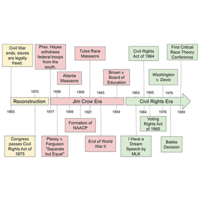
A review and critique of critical race theory and feminist standpoint theory while providing guidance for using these theories in physics education research.
Eye tracking in physics education research: A systematic literature review
L. hahn and p. klein, phys. rev. phys. educ. res. 18 , 013102 (2022) – published 2 march 2022.
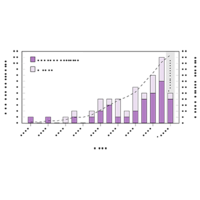
The use of eye tracking in physics education research is growing rapidly and has led to insights into students’ learning processes.
Sign up to receive regular email alerts from Physical Review Physics Education Research
- Forgot your username/password?
- Create an account
Article Lookup
Paste a citation or doi, enter a citation.
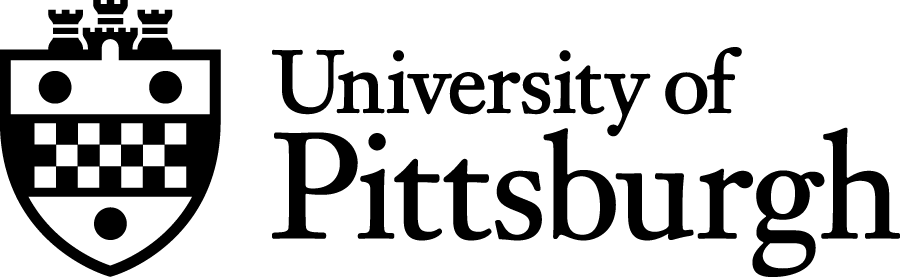
- open search
From the latest big breakthrough to the most influential and inspiring figures on campus to Pitt in the community, Pittwire is your official source for what’s happening now.
- Health and Wellness
- Technology and Science
- Arts and Humanities
- Community Impact
- Diversity, Equity, and Inclusion
- Innovation and Research
- Our City/Our Campus
- Pitt Magazine
- Features & Articles
- Accolades & Honors
- Ones to Watch
- Announcements and Updates
- Life at Pitt
- Arts & Sciences
- Computing & Information
- Dental Medicine
- Engineering
- General Studies
- Health & Rehabilitation
- Honors College
- Public & Intl Affairs
- Public Health
- Social Work
- COVID-19 Response
- Sustainability
- Teaching & Learning
- Kenneth P. Dietrich School of Arts and Sciences
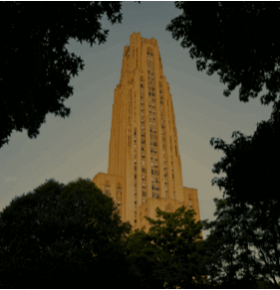
Subscribe to Pittwire Today
Chandralekha singh received an aapt award for physics education.
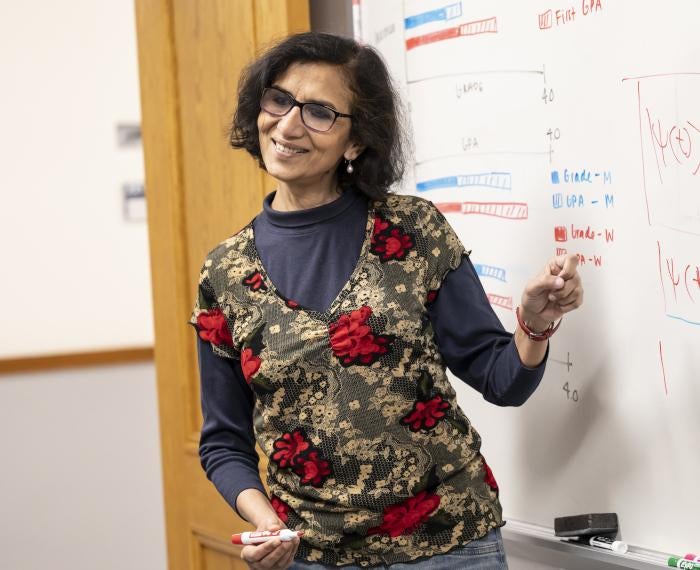
The University of Pittsburgh’s Chandralekha Singh , a distinguished professor of physics in the Kenneth P. Dietrich School of Arts and Sciences, has been named the John David Jackson Excellence in Graduate Physics Education Award winner for 2024, the American Association of Physics Teachers announced.
The award is presented to physicists and physics educators who have made outstanding contributions to curriculum development, mentorship or classroom teaching in graduate physics education.
“I am truly honored and humbled to get this award,” said Singh in an AAPT press release . “I have been a member of AAPT since the late ’ 90s, and I am incredibly grateful to AAPT for providing this stimulating community of educators who are passionate about improving the teaching and learning of physics at all levels.”
Singh earned the recognition for her education research work, which has had a wide-reaching impact on graduate physics education and scholarship, along with her own skilled teaching and mentoring practices.
Singh’s work on teaching and learning of quantum mechanics has played a substantial role in pioneering advancements in the greater field of physics education research in advanced courses. She has also conducted research on cognitive issues in learning physics, improving student problem solving and reasoning skills as well as equity and inclusion in physics learning environments.
The award will be presented at the 2024 Summer Meeting of the AAPT, to be held July 6-10 in Boston.
— Nick France, photography by Aimee Obidzinski
Pitt is updating its Campus Master Plan
Employees, benefits open enrollment is may 1-15, pitt is launching an office of sustainability in the health sciences.
The request to the URL needs to be verified.
The request to the URL is paused, and must be verified for you to access it. This question is for testing whether you are a human visitor, and to prevent automated spam submission.
What code is in the image submit
Incident ID: 14604593417942947546
For comments and questions: [email protected]
- Contact Us!
Department of Physics
You are here, gabriel t assumpção awarded the 2023-2024 d. allan bromley graduate fellowship in physics.
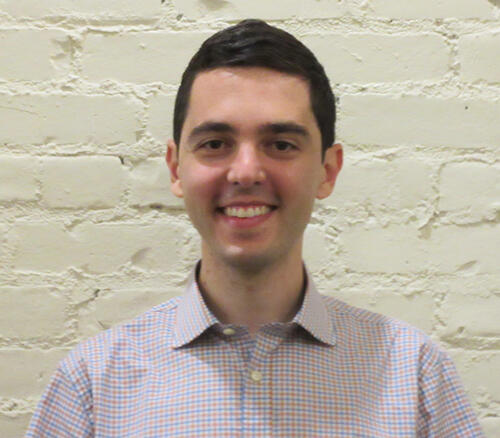
Gabriel T Assumpção is the recipient of this year’s Yale Physics D. Allan Bromley Graduate Fellowship in Physics .
Assumpção’s citation reads, “ For his unparalleled dedication and passion for teaching and mentoring in the context of building experimental skills at many levels: in outreach, in the instructional labs of Yale college and in the research lab .”
Gabriel G. T. Assumpção is a sixth-year graduate student working with Prof. Nir Navon.
As member of the first generation of graduate students in Prof. Navon’s lab, Gabriel helped design and build the group’s intricate experimental setup in the basement of SPL and has since been an avid mentor to new generations of graduate student, transferring the unwritten experimental skills necessary to build, run, and upkeep the complex custom-built machinery. Gabriel has also supervised a handful of undergraduates, devising projects in the lab that aligned to students’ diverse learning goals, and that developed proficiency and fostered a sense of responsibility.
Gabriel’s interest in teaching experimental techniques extended to the four semesters he was a teaching fellow for the advanced physics laboratory class. Besides teaching students, he extensively checked and debugged several experiments at least a month before each semester started, and kept many setups operational while the class was running. Previous to that, Gabriel was a leading TF for the General Physics Laboratory, editing and updating old rubrics, as well as enthusiastically teaching non-physics-major students, for which he got superb evaluations.
Throughout his time at Yale, Gabriel has also supplemented his interest in mentoring and teaching by pursuing courses at the Poorvu Center, including programs in mentoring in the lab and effective scientific teaching. Finally, in community outreach, whenever available Gabriel has been a volunteer with the Yale Physics Olympiad (YSO) and the Girls’ Science Investigations (GSI) programs.
The D. Allan Bromley Fellowship Fund for Graduate Research in Physics was established in 2005 to honor Professor D. Allan Bromley, Sterling Professor of the Sciences at Yale University and former Presidential Science Advisor to President George H. W. Bush. The fellowship was created by Bromley’s former students Joel Birnbaum (Yale Physics PhD ‘65), Joe Allen (Yale Physics PhD ‘65), and John Manoyan (Yale Physics PhD ‘87), because of their “deep affection and respect for” Bromley, in order to “memorialize his exceptional teaching and research career at Yale.”
The fellowship is awarded annually to graduate students in physics who have advanced to candidacy in the Ph.D. program, particularly those “who exhibit a broader interest than just physics, including, but not limited to, science and public policy, engineering, and applied science.” Candidates are nominated by the Physics faculty and selected by the D. Allan Bromley Professor of Physics (currently Keith Baker), the Director of Graduate Studies (currently Daisuke Nagai) and Chair (Karsten Heeger). The description continues, “In this way, the recipients will reflect and celebrate Dr. Bromley’s distinguished and honorable persona in the exceptional scope, standing, talent, and character of his distinguished personal, public, and academic life.”
- Prospective students
- International students
- PhD candidates
- Professors and researchers
- Institutions and companies
- Academic Board
- Career opportunities
Research topics
- Advisory Board
- Regulations
Here are the four major areas where one can find research proposals for a PhD thesis in Physics:
Experimental Physics of Matter
Magnetization dynamics in thin films and nanostructures
Investigation of magnetization processes of bulk superconducting materials for the development of devices for shielding and production/optimization of magnetic fields
Control of the properties of two-dimensional materials by electric field effect
Experimental investigation of the order parameter in unconventional superconductors
Quantum technologies in the frame of quantum optics
Evaluation of the therapeutic and imaging properties of stimuli-activated semiconducting nanocrystals against cancer cells
Memristors and Mechanical resonators
Materials and processes for micro & nano technologies
Modeling and experiments of elastic wave propagation in complex media and acoustic/elastic metamaterials
NanoPhotonics for Quantum Optics
Theoretical Physics of Matter
Microscopic theory and simulation of electronic quantum nanodevices
Disorder operators and topological orders in strongly correlated quantum matter
Quantum mixture formed by two bosonic components
Numerical Monte Carlo simulation of strongly correlated electronic models
Out of equilibrium properties of quantum systems: investigation of topological materials
Exploring the power of Entanglement for information processing: quantum information theory for quantum technologies
Interaction of mesoscopic quantum systems with gravity
Physics of Complex Systems
Non equilibrium Statistical Mechanics
Statistical physics of the living cell
High Energy Physics
Supergravity and String Theory
Nuclear matter under extreme conditions and high energy astrophysics
Senior Staff Scientist
Marisa hughes.
Dr. Marisa Hughes is the assistant program manager for Human and Machine Intelligence in APL’s Research and Exploratory Development Mission Area. Her focus is in artificial intelligence (AI), especially as it applies to climate intelligence. This includes AI methods to detect greenhouse gas emissions, assess climate tipping points, augment environmental sensing, and enhance operational forecasts of dynamic Earth systems. Dr. Hughes is the founder of the APL Climate Network and co-leads a Laboratory-wide initiative addressing the national and global challenges resulting from climate change. Additional areas of expertise include systems interaction, graph analytics, mathematics, data science, and modeling .
Ph.D., Mathematics
Cornell University
M.S., Mathematics
B.s., mathematics.
Binghamton University
Mission Area
- Research and Exploratory Development
PROGRAMS SUPPORTED
- Human and Machine Intelligence
- Biological and Chemical Sciences
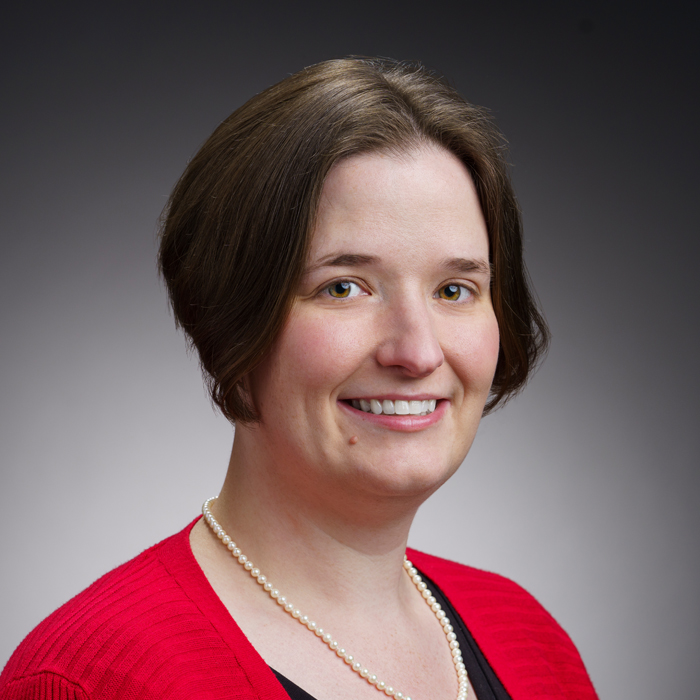
This website uses cookies to measure traffic and improve your experience. View our Privacy Policy to learn more.

- Follow us on Facebook
- Follow us on Twitter
- Follow us on LinkedIn
- Watch us on Youtube
- Latest Explore all the latest news and information on Physics World
- Research updates Keep track of the most exciting research breakthroughs and technology innovations
- News Stay informed about the latest developments that affect scientists in all parts of the world
- Features Take a deeper look at the emerging trends and key issues within the global scientific community
- Opinion and reviews Find out whether you agree with our expert commentators
- Interviews Discover the views of leading figures in the scientific community
- Analysis Discover the stories behind the headlines
- Blog Enjoy a more personal take on the key events in and around science
- Physics World Live
- Impact Explore the value of scientific research for industry, the economy and society
- Events Plan the meetings and conferences you want to attend with our comprehensive events calendar
- Innovation showcases A round-up of the latest innovation from our corporate partners
- Collections Explore special collections that bring together our best content on trending topics
- Artificial intelligence Explore the ways in which today’s world relies on AI, and ponder how this technology might shape the world of tomorrow
- #BlackInPhysics Celebrating Black physicists and revealing a more complete picture of what a physicist looks like
- Nanotechnology in action The challenges and opportunities of turning advances in nanotechnology into commercial products
- The Nobel Prize for Physics Explore the work of recent Nobel laureates, find out what happens behind the scenes, and discover some who were overlooked for the prize
- Revolutions in computing Find out how scientists are exploiting digital technologies to understand online behaviour and drive research progress
- The science and business of space Explore the latest trends and opportunities associated with designing, building, launching and exploiting space-based technologies
- Supercool physics Experiments that probe the exotic behaviour of matter at ultralow temperatures depend on the latest cryogenics technology
- Women in physics Celebrating women in physics and their contributions to the field
- Audio and video Explore the sights and sounds of the scientific world
- Podcasts Our regular conversations with inspiring figures from the scientific community
- Video Watch our specially filmed videos to get a different slant on the latest science
- Webinars Tune into online presentations that allow expert speakers to explain novel tools and applications
- IOP Publishing
- Enter e-mail address
- Show Enter password
- Remember me Forgot your password?
- Access more than 20 years of online content
- Manage which e-mail newsletters you want to receive
- Read about the big breakthroughs and innovations across 13 scientific topics
- Explore the key issues and trends within the global scientific community
- Choose which e-mail newsletters you want to receive
Reset your password
Please enter the e-mail address you used to register to reset your password
Registration complete
Thank you for registering with Physics World If you'd like to change your details at any time, please visit My account
- Education and outreach
Data science CDT puts industry collaboration at its heart
The Liverpool Centre for Doctoral Training (CDT) for Innovation in Data Intensive Science is bridging disciplines and empowering PhD students to excel in data-intensive research in both industry and academia
Physics is a constantly evolving field – how do we make sure the next generation of physicists receive training that keeps pace with new developments and continues to support the cutting edge of research?
According to Carsten P Welsch , a distinguished accelerator scientist at the University of Liverpool , in the age of machine learning and AI, PhD students in different physics disciplines have more in common than they might think.
“Research is increasingly data-intensive, so while a particle physicist and a medical physicist might spend their days thinking about very different concepts, the approaches, the algorithms, even the tools that people use, are often either the same or very similar,” says Professor Welsch.
Data science is extremely important for any type of research and will probably outlive any particular research field Professor Welsch
Welsch is the director of the Liverpool Centre for Doctoral Training (CDT) for Innovation in Data Intensive Science (LIV.INNO). Founded in 2022, the CDT is currently recruiting its third cohort of PhD students. Current students are undertaking research that spans medical, environmental, particle and nuclear physics, but their projects are all underpinned by data science. According to Professor Welsch, “Data science is extremely important for any type of research and will probably outlive any particular research field.”
Next-generation PhD training
Carsten Welsch has a keen interest in improving postgraduate education, he was chair of STFC’s Education Training and Careers Committee and a member of the UKRI Skills Advisory Group. When it comes to the future of doctoral training he says “The big question is ‘where do we want UK researchers to be in a few years, across all of the different research areas?’”
He believes that LIV.INNO holds the solution. The CDT aims to give students with data-intensive PhD projects the skills that will enable them to succeed not only in their research but throughout their careers.
Lauryn Eley is a PhD student in the first LIV.INNO cohort who is researching medical imaging. She became interested in this topic during her undergraduate studies because it applied what she had learned in university to real-world situations. “It’s important that I can see the benefits of my work translated into everyday experiences, which I think medical imaging does quite nicely,” she says.
Miss Eley’s project is partnered with medical technology company Adaptix . The company has developed a mobile X-ray device which, it hopes, will enable doctors to produce a high-quality 3D X-ray image more cheaply and easily than with a traditional CT scanner.
Her task is to build a computational model of the X-ray device and investigate how to optimize the images it produces. To generate high-quality results she must simulate millions of X-rays. She says that the data science training she received at the start of the PhD has been invaluable.
From their first year, students attend lectures on data science topics which cover Monte Carlo simulation, high-performance computing, machine learning and AI, and data analysis. Lauryn Eley has an experimental background, and she says that the lectures enabled her to get to grips with the C++ she needed for her research.
Boosting careers with industry placements
Professor Welsch says that from the start, industry partnership has been at the centre of the LIV.INNO CDT. Students spend six months of their PhD on an industrial placement, and Lauryn Eley says that her work with Adaptix has been eye-opening, enabling her to experience first-hand the fast-paced, goal-driven world of industry, which she found very different to academic research.
While the CDT may particularly appeal to those keen on pursuing a career in industry, Professor Welsch emphazises the importance of students delivering high-quality research. Indeed, he believes that LIV.INNO’s approach provides students with the best chance of success in their academic endeavours. Students are taught to use project management skills to plan and deliver their projects, which he says puts them “in the driving seat” as researchers. They are also empowered to take initiative, working in partnership with their supervisors rather than waiting for external guidance.
LIV.INNO builds on a previous programme called the Liverpool Big Data Science Centre for Doctoral Training, which ran between 2017 and 2024. Professor Welsch was also the director of that CDT, and he has noticed that when it comes to partnering with student projects, industry attitudes have undergone a shift.
“When we approached the companies for the first time, you could definitely see that there was a lot of scepticism,” he says. “However, with the case studies from the first CDT, they found it much easier to attract industry partners to LIV.INNO.” Professor Welsch thinks that this demonstrates the benefits that industry-academia partnerships bring to both students and companies.
The first cohort from LIV.INNO are only in their second year, but many of the students from the previous CDT secured full-time jobs from the company where they did their placement. But whatever career path students eventually go down, Carsten Welsch is convinced that the cross-sector experience students get with LIV.INNO sets them up for success, saying “They can make a much better informed decision about where they would like to continue their careers.”

Want to read more?
- E-mail Address
Sponsored by Liverpool Centre for Doctoral Training (CDT)
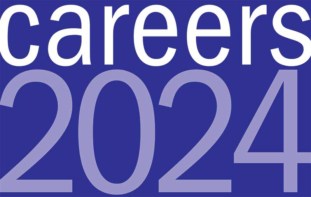
Physics World Careers
Providing valuable careers advice and a comprehensive employer directory
- Particle and nuclear
‘My career has not been a straight line’: Craig Jantzen on switching from nuclear science to diplomacy
Discover more from physics world.
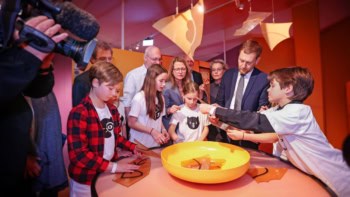
Quantum-themed escape room opens at German science museum

Peter Higgs: Nobel-prize-winning particle theorist dies aged 94

Related jobs
Helping you train to teach physics, external dosimetry health physicist, lead threat intelligence analyst – leeds – national security, related events.
- Materials | Symposium The Eigthth International Symposium on Dielectric Materials and Applications (ISyDMA'8) 12—16 May 2024 | Orlando, US
- Materials | Symposium Eighth International Symposium on Dielectric Materials and Applications (ISyDMA'8) 12—16 May 2024 | Orlando, US
- Biophysics and bioengineering | Workshop Chemotaxis – from Basic Physics to Biology 13—17 May 2024 | Dresden, Germany

- research associate department physics
Research Associate - Department of Physics
Research Associate, Soft matter, biophysics, and expansion microscopy Department of Physics
The Department of Physics at Case Western Reserve University College of Arts and Sciences seeks one staff Research Associate to conduct research in the laboratory of Prof. Lydia Kisley starting as soon as the candidate is available. The ideal candidate will work at the interface of biophysics, biomaterials, and analytical chemistry developing a method to detect nutrients at subcellular resolutions. Overall, the Kisley lab studies soft materials using single-molecule microscopy with the goal to advance the single-molecule materials field towards more complex, realistic conditions. More information on the broad focus of the lab can be found at http://physics.case.edu/faculty/lydia-kisley/
The candidate will primarily be responsible for working on a project funded by a private foundation to develop a super-resolution microscopy method using novel soft hydrogel materials. It is required that the candidate will have extensive experience with hydrogels and characterizing soft materials. Further skills in in eukaryotic cell culture, fluorescence microscopy, image processing in MATLAB, Python, or other C-based programming languages are preferred. It is not required, but also ideal, if the candidate has experience in extracellular matrix/mechanobiology or MALDI mass spectrometry. The project is highly collaborative with Prof. Laura Sanchez’ bioanalytical chemistry research group at UC Santa Cruz and opportunities for travel are included in this position. Finally, the Research Associate in their staff role will also support the lab environment by managing ordering of supplies and maintaining updates to the group website.
The candidate will be responsible for working with postdoctoral researchers, staff, graduate, and undergraduate students in the lab and participate in maintaining a strong lab culture within the research group. Regular responsibilities will be generating data and text for progress reports, manuscripts and grants, participating in weekly meetings, and assisting with training and mentoring of lab members. In addition, the lab will provide career development opportunities for the candidate such as funds for annual national conference travel and support in developing pedagogical skills and grantsmanship, dependent on the candidate’s future career interests. More details about the lab culture and mentoring approach can be found at: https://www.kisleylab.science/lab-manual-mentoring-approach
S upport for this position is available for 2 years above NIH NRSA Stipend Levels and includes benefits, conditional on annual reviews. There is the potential for the position to be extended longer dependent on funding levels in the lab and for future promotion to either Senior Research Associate or a Research Assistant Professor position dependent on the candidate’s skills and interest in independent grant writing. Sponsorship for a visa is available, if required.
The Department of Physics at CWRU is part of a strong community of soft matter and biophysics research and activities in the Cleveland area. Possibilities to collaborate and network within CWRU School of Medicine, School of Engineering departments such as Macromolecular Sciences and Engineering, Biomedical Engineering, and Mechanical Engineering Cleveland Clinic, University Hospitals, among others, exist in this position.
The Kisley Research Group believes that the best research results from working with a diverse team. We foster a sense of community in our group where everyone should feel welcome, respected, and safe. We strongly encourage applications from female and minority candidates, along with those who have taken efforts to improve diversity in STEM. More information on our philosophy can be found at: https://www.kisleylab.science/diversity-outreach
The applicant must have a Ph.D. degree in Experimental Biophysics, Polymer Science, Bioengineering, Mechanical Engineering, Biophysical Chemistry, or a similar field. Interested individuals should send a cover letter, CV, a representative publication in PDF form, and the contact information of three people who could provide recommendations to [email protected].
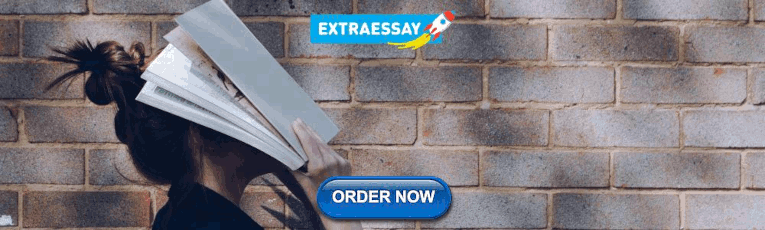
IMAGES
VIDEO
COMMENTS
August 4, 2021. Physics Education Research (PER) uses various research methods classified under qualitative, quantitative, and mixed methods. These approaches help researchers understand physics education phenomena and advance our efforts to produce better PER. Over time, research questions and contexts have evolved, and so have our methods.
Physics Education. ISSN: 1361-6552. SUPPORTS OPEN ACCESS. Physics Education is the international journal for everyone involved with the teaching of physics in schools and colleges. The articles reflect the needs and interests of secondary school teachers, teacher trainers and those involved with courses up to introductory undergraduate level.
Using evidence-based approaches to improve the teaching of physics can help students achieve more and improve equity. In this Focus Issue, we survey the current state of this research field.
Physics Education Research (PER) is the study of how people learn physics and how to improve the quality of physics education. Researchers use the tools and methods of science to answer questions about physics learning that require knowledge of physics. Researchers focus on developing objective means of measuring the outcomes of educational ...
The edited book, Teaching-Learning Contemporary Physics: from Research to Practice (Jarosievitz & Sükösd, 2021), is part of Springer's "Challenge in Physics Education" series addressed to professional readers including researchers, teachers, instructional designers, and pre-service teachers.The book comprises five parts including 19 chapters that clearly explain contemporary physics ...
Paula R. L. Heron, Ph.D., is Professor of Physics at the University of Washington where she has been teaching since 1995.She is co-founder and co-chair of the biannual "Foundations and Frontiers in Physics Education Research" conference series, and she has been a committee executive for groups within the American Physical Society (APS), American Association of Physics Teachers (AAPT), and ...
Education goals have evolved to emphasize student acquisition of essential "21 st Century skills", which define the knowledge and attributes necessary to successfully contribute to the workforce and global economy of the 21st Century (National Research Council, 2011, 2012a).In general, these standards seek to transition from emphasizing content-based drilling and memorization towards ...
By Abigail Dove. The APS Topical Group on Physics Education Research (GPER) is a home for members interested in research on the learning and teaching of physics—a broad, multidisciplinary area encompassing everything from institutional practices to neural and cognitive processes to the social and contextual components of physics educational ...
Physics faculty—experts in evidence-based research—often rely on anecdotal experience to guide their teaching practices. Adoption of research-based instructional strategies 4 remains surprisingly low, despite a large body of physics education research (PER) spanning over two decades, and extensive dissemination efforts by physics education researchers and innovators (Turpen and Finkelstein ...
Many research-based teaching methods in physics, including Peer Instruction, CAE Think-Pair-Share, Technology Enhanced Formative Assessment, and teaching with clickers, involve having your students discuss and answer multiple-choice conceptual questions. A challenge of using these methods is finding and writing good questions.
Physics Education Research. The CERN Convention cites engaging the public with fundamental research as a vital part of the Organization's mission. Indeed, CERN and the experimental collaborations it hosts have a considerable history of high-quality communication and outreach efforts that reach a wide range of international audiences.
As the field of physics education research grows, it is increasingly difficult for newcomers to gain an appreciation of the major findings across all sub-domains, discern global themes, and recognize gaps in the literature. The current volume incorporates the understanding of both physics and education concepts and provides an extensive review ...
Physics Education Research focuses on improving the teaching of physics by understanding the cognitive mechanisms which either aid or hinder student learning, and developing pedagogical techniques to improve learning. Chandralekha Singh has led numerous studies documenting student difficulties in both introductory and advanced physics classes ...
Physics education research (PER) is a form of discipline-based education research specifically related to the study of the teaching and learning of physics, often with the aim of improving the effectiveness of student learning.PER draws from other disciplines, such as sociology, cognitive science, education and linguistics, and complements them by reflecting the disciplinary knowledge and ...
Physics and Science Education Research. The Wieman group's research falls into the following main categories: Uses of PhET Simulations across the K-16 curriculum. PhET simulations (phet.colorado.edu) are interactive science simulations that are extensively used by a wide range of students and teachers (around 100 million uses this year in a ...
Physics Education Research (PER) works toward theoretical understanding and practical advancement of instruction, specifically concerned with the disciplinary knowledge and practices of physics. Researchers work within and contribute to the PER community, situated mainly in departments of physics, as well as the (much larger) communities of the ...
Research Instruments. The Symmetry and Gauss's Law Conceptual Evaluation, developed by Chandralekha Singh, is a research-based multiple-choice test designed to evaluate students' conceptual understanding of symmetry and Gauss's Law. The test is appropriate for research in calculus-based introductory physics courses or upper-level undergraduate ...
strategies to improve teaching and learning in Fundamental Physics cou rse" lists four. strategies in teaching physics: problem based learning, concept mapping, team work. and mini-lecture and ...
Research and Outreach. Physics Education Research at UCF is led by two tenure-track assistant professors. Both Dr. Jackie Chini and Dr. Zhongzhou Chen received their Ph.D.s in physics for research in physics education. We study issues relevant to the evolving landscape of higher education in physics and collaborate with colleagues in related ...
Phys. Rev. Phys. Educ. Res. 18, 010136 (2022) - Published 2 May 2022. Retrieval practice of physics principles and their conditions of application before self-explanation can have a positive effect on posttest problem-solving scores and can increase the quality of students' self-explanations.
The University of Pittsburgh's Chandralekha Singh, a distinguished professor of physics in the Kenneth P. Dietrich School of Arts and Sciences, has been named the John David Jackson Excellence in Graduate Physics Education Award winner for 2024, the American Association of Physics Teachers announced.. The award is presented to physicists and physics educators who have made outstanding ...
Department of Physics and Astronomy Physics Education Research Uppsala University Box 516 SE-751 20 Uppsala Sweden. Visiting Address. Ångström Laboratory, Building 9, floor 1 Lägerhyddsvägen 1, Uppsala. Deliveries. Regementsvägen 1 SE-752 37 Uppsala. Telephone. 018-471 0000. Fax +46 18 471 3524
Gabriel T Assumpção is the recipient of this year's Yale Physics D. Allan Bromley Graduate Fellowship in Physics.. Assumpção's citation reads, "For his unparalleled dedication and passion for teaching and mentoring in the context of building experimental skills at many levels: in outreach, in the instructional labs of Yale college and in the research lab."
Education. Education Toggle submenu. Education. Go to page Education. Applying, studying, graduating; ... Physics; Research topics Research topics. Here are the four major areas where one can find research proposals for a PhD thesis in Physics: Experimental Physics of Matter ...
Dr. Marisa Hughes is the assistant program manager for Human and Machine Intelligence in APL's Research and Exploratory Development Mission Area. Her focus is in artificial intelligence (AI), especially as it applies to climate intelligence. This includes AI methods to detect greenhouse gas emissions, assess climate tipping points, augment environmental sensing, and enhance operational ...
Current students are undertaking research that spans medical, environmental, particle and nuclear physics, but their projects are all underpinned by data science. According to Professor Welsch, "Data science is extremely important for any type of research and will probably outlive any particular research field." Next-generation PhD training
And much of the groundbreaking findings from the university are coming from researchers in the College of Letters & Science. With experts in fields ranging from computer science and physics to journalism and history, we are at the forefront of research that answers some of the toughest questions facing our society today.
Research Associate, Soft matter, biophysics, and expansion microscopy Department of Physics The Department of Physics at Case Western Reserve University College of Arts and Sciences seeks one staff Research Associate to conduct research in the laboratory of Prof. Lydia Kisley starting as soon as the candidate is available.