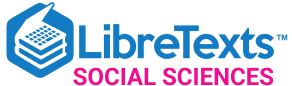
- school Campus Bookshelves
- menu_book Bookshelves
- perm_media Learning Objects
- login Login
- how_to_reg Request Instructor Account
- hub Instructor Commons
- Download Page (PDF)
- Download Full Book (PDF)
- Periodic Table
- Physics Constants
- Scientific Calculator
- Reference & Cite
- Tools expand_more
- Readability
selected template will load here
This action is not available.
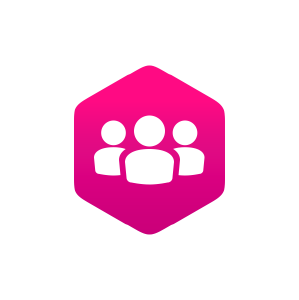
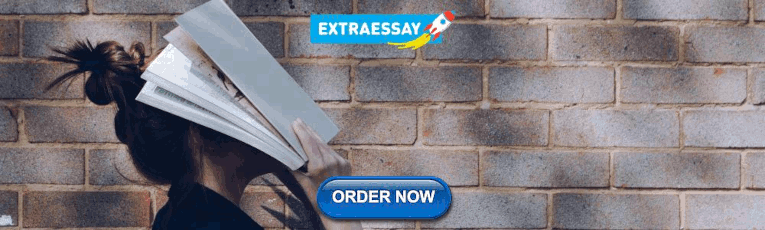
36.1: Introduction to Natural Resource Economics
- Last updated
- Save as PDF
- Page ID 4789
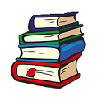
Types of Natural Resources
- Natural resource economics focuses on the supply, demand, and allocation of the Earth’s natural resources.
learning objectives
- Analyze natural resource economics and explain the types of natural resources that exist.
Natural Resource Economics
Natural resource economics focuses on the supply, demand, and allocation of the Earth’s natural resources. It’s goal is to gain a better understanding of the role of natural resources in the economy. Learning about the role of natural resources allows for the development of more sustainable methods to manage resources and make sure that they are maintained for future generations.The goal of natural resource economics is to develop an efficient economy that is sustainable in the long-run.
Importance of the Environment : This diagram illustrates how society and the economy are subsets of the environment. It is not possible for societal and economic systems to exist independently from the environment. For this reason, natural resource economics focuses on understanding the role of natural resources in the economy in order to develop a sufficient and sustainable economy that protects natural resources.
Natural resources are derived from the environment. Some of the resources are essential to survival, while others merely satisfy societal wants. Every man-made product in an economy is composed of natural resources to some degree.
There are numerous ways to classify the types of natural resources, they include the source of origin, the state of development, and the renewability of the resources.
In terms of the source of origin, natural resources can be divided into the following types:
- Biotic: these resources come from living and organic material, such as forests and animals, and include the materials that can be obtained them. Biotic natural resources also include fossil fuels such as coal and petroleum which are formed from organic matter that has decayed.
- Abiotic: these resources come from non-living and non-organic material. Examples of these resources include land, fresh water, air, and heavy metals (gold, iron, copper, silver, etc.).
Natural resources can also be categorized based on their stage of development including:
- Potential resources: these are resources that exist in a region and may be used in the future. For example, if a country has petroleum in sedimentary rocks, it is a potential resource until it is actually drilled out of the rock and put to use.
- Actual resources: these are resources that have been surveyed, their quantity and quality has been determined, and they are currently being used. The development of actual resources is dependent on technology.
- Reserve resources: this is the part of an actual resource that can be developed profitably in the future.
- Stock resources: these are resources that have been surveyed, but cannot be used due a lack of technology. An example of a stock resource is hydrogen.
Natural resources are also classified based on their renewability:
- Renewable natural resources: these are resources that can be replenished. Examples of renewable resources include sunlight, air, and wind. They are available continuously and their quantity is not noticeably affected by human consumption. However, renewable resources do not have a rapid recovery rate and are susceptible to depletion if they are overused.
- Non-renewable natural resources: these resources form extremely slow and do not naturally form in the environment. A resource is considered to be non-renewable when their rate of consumption exceeds the rate of recovery. Examples of non-renewable natural resources are minerals and fossil fuels.
There is constant worldwide debate regarding the allocation of natural resources. The discussions are centered around the issues of increased scarcity (resource depletion) and the exportation of natural resources as a basis for many economies (especially developed nations). The vast majority of natural resources are exhaustible which means they are available in a limited quantity and can be used up if they are not managed correctly. Natural resource economics aims to study resources in order to prevent depletion.
Natural resource utilization is regulated through the use of taxes and permits. The government and individual states determine how resources must be used and they monitor the availability and status of the resources. An example of natural resource protection is the Clean Air Act. The act was designed in 1963 to control air pollution on a national level. Regulations were established to protect the public from airborne contaminants that are hazardous to human health. The act has been revised over the years to continue to protect the quality of the air and health of the public in the United States.
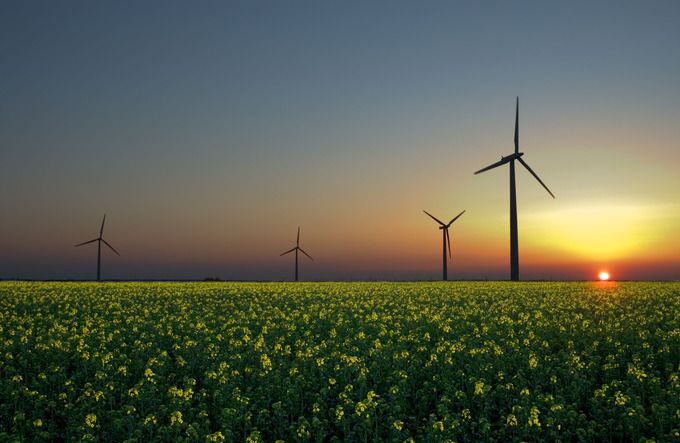
Wind : Wind is an example of a renewable natural resource. It occurs naturally in the environment and has the ability to replenish itself. It has also been used as a form of energy development through wind turbines.
Basic Economics of Natural Resources
Natural resource economics focuses on the supply, demand, and allocation of the Earth’s natural resources to create a more efficient economy.
- Explain basic natural resource economics
Natural resource economics focuses on the supply, demand, and allocation of the Earth’s natural resources. The main objective of natural resource economics is to gain a better understanding of the role of natural resources in the economy. By studying natural resources, economists learn how to develop more sustainable methods of managing resources to ensure that they are maintained for future generations. Economists study how economic and natural systems interact in order to develop an efficient economy.
As a field of academic research, natural resource economics addresses the connections and interdependence between human economies and natural ecosystems. The focus is how to operate an economy within the ecological constraints of the earth’s natural resources.
Natural Resource Economics : This diagram illustrates that society and the economy are subsets of the environment. It is not possible for social and economic systems to exist independently from the environment. Natural resource economics focuses on the demand, supply, and allocation of natural resources to increase sustainability.
Areas of Study
Economists study the commercial and recreational use and exploitation of resources. Traditionally, natural resource economics focused on fishery, forestry, and mineral models. However, in recent years many more topics have become increasingly important, including air, water, and the global climate. Natural resource economics is studied on an academic level, and the findings are used to shape and direct policy-making for environmental issues.
Examples of areas of study in natural resource economics include:
- welfare theory
- pollution control
- resource exhaustibility
- environmental management
- resource extraction
- non-market valuation
- environmental policy
Additionally, research topics of natural resource economists can include topics such as the environmental impacts of agriculture, transportation and urbanization, land use in poor and industrialized countries, international trade and the environment, and climate change.
Impact of Natural Resource Economics
The findings of natural resource economists are used by governments and organizations to better understand how to efficiently use and sustain natural resources. The findings are used to gain insight into the following environmental areas:
- Extraction: the process of withdrawing resources from nature. Extractive industries are a basis for the primary sector of the economy. The extraction of natural resources substantially increases a country’s wealth. Economists study extraction rates to make sure that resources are not depleted. Also, if resources are extracted too quickly, the sudden inflow of money can cause inflation. Economists seek to maintain a sense of balance within extraction industries.
- Depletion: the using up of natural resources, which is considered to be a global sustainable development issue. Many governments and organizations have become increasingly involved in preserving natural resources. Economists provide data to determine how to balance the needs of societies now and preserve resources for the future.
- Protection: the preservation of natural resources for the future. The findings of economists help governments and organization develop measures of protection to sustain natural resources. Protection policies state the necessary actions internationally, nationally, and individually that must take place to control natural resource depletion that is a result of human activity.
- Management: the use of natural resources taking into account economic, environmental, and social concerns. This process deals with managing natural resources such as land, water, soil, plants, and animals. Particular focus is placed on how the preservation of natural resources impacts the quality of life now and for future generations.
Externalities and Impacts on Resource Allocation
Production and use of resources can have a positive or negative effect on the allocation of the natural resources.
- Examine externalities and how they the impact resource allocation of natural resources.
Resource Allocation
Resource allocation is division of goods for the use of production within the economy. The needs and wants of society as well as industries impact what is produced. Suppliers focus on producing the varieties of goods and services that will yield the greatest satisfaction to consumers. In the long run, externalities directly impact resource allocation. It must be determined whether the production, as well as the process of production, creates more benefits that costs for the producers, consumers, and society as a whole.
Externalities
An externality is a cost or benefit that affects a party who did not choose to incur the cost or benefit. In regards to natural resources, production and use of resources can have a positive or negative effect on the allocation of the resources.
External Costs
A negative externality, also called the external cost, imposes a negative effect on a third party to an economic transaction. Many negative externalities impact natural resources negatively because of the environmental consequences of production and use. For example, air pollution from factories and vehicles can cause damage to crops. Likewise, water pollution has a negative impact of plants and animals.
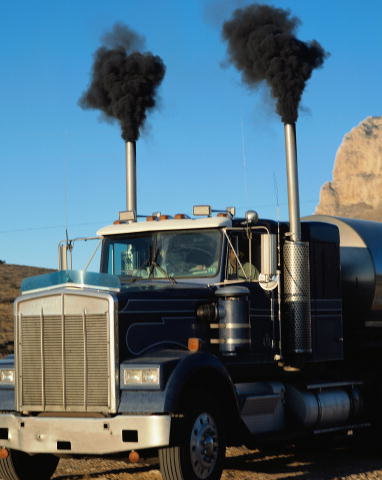
Negative externality : Air pollution from vehicles is an example of a negative externality. It affects other than those who drive the vehicle and those who sell the gas.
In the case of negative externalities, the marginal private cost of consuming a good is less than the marginal social or public cost. The marginal social benefit should equal the marginal social cost (i.e. production should only be increased when the marginal social benefit exceeds the marginal social cost). When external costs are present, the use of natural resources is inefficient because the social benefit is less than the social cost. In other words, society and the natural resources involved would have been better off if the natural resources had not been used at all.
Developed countries use more natural resources and must enact sustainable development plan for the use of resources. Human needs must be met, but the environment and natural resources must be preserved. Examples of resource depletion include mining, petroleum extraction, fishing, forestry, and agriculture.
External Benefits
Positive externalities, also referred to as external benefits, impose a positive effect on a third party. An example of a positive externality is when crops are pollinated by bees from a neighboring bee farm. In order to achieve the socially optimal equilibrium, the marginal social benefit should equal the marginal social cost (i.e. production should be increased as long as the marginal social benefit exceeds the marginal social cost). Assuming that natural resources are used and also sustained, the external benefits of goods produced by natural resources impacts the majority of the public in a positive way.
- Every man-made product in an economy is composed of natural resources to some degree.
- Natural resources can be classified as potential, actual, reserve, or stock resources based on their stage of development.
- Natural resources are either renewable or non-renewable depending on whether or not they replenish naturally.
- Natural resource utilization is regulated through the use of taxes and permits. The government and individual states determine how resources must be used and they monitor the availability and status of the resources.
- As a field of academic research, natural resource economics addresses the connections and interdependence between human economies and natural ecosystems.
- By studying natural resources, economists learn how to develop more sustainable methods of managing resources to ensure that they are maintained for future generations.
- Natural resource economics is studied on an academic level, and the findings are used to shape and direct policy-making for environmental issues. These issues include resource extraction, depletion, protection, and management.
- Natural resource economics findings impact policies for environmental work including issues such as extraction, depletion, protection, and management.
- An externality is a cost or benefit that affects a party who did not choose to incur the cost or benefit.
- A negative externality, also called the external cost, imposes a negative effect on a third party.
- When external costs are present, the market equilibrium use of natural resources is inefficient because the social benefit is less than the social cost. In other words, society would have been better off if fewer natural resources had been used.
- Positive externalities, also referred to as external benefits, imposes a positive effect on a third party.
- Assuming that natural resources are used and also sustained, the external benefits of goods produced by natural resources impacts the majority of the public in a positive way.
- natural resource : Any source of wealth that occurs naturally, especially minerals, fossil fuels, timber, etc.
- Renewable : Sustainable; able to be regrown or renewed; having an ongoing or continuous source of supply; not finite.
- depletion : The consumption of a resource faster than it can be replenished.
- sustainable : Able to be sustained for an indefinite period without damaging the environment, or without depleting a resource.
- externality : An impact, positive or negative, on any party not involved in a given economic transaction or act.
LICENSES AND ATTRIBUTIONS
CC LICENSED CONTENT, SHARED PREVIOUSLY
- Curation and Revision. Provided by : Boundless.com. License : CC BY-SA: Attribution-ShareAlike
CC LICENSED CONTENT, SPECIFIC ATTRIBUTION
- Boundless. Provided by : Boundless Learning. Located at : www.boundless.com//political-...tion/renewable . License : CC BY-SA: Attribution-ShareAlike
- Natural resource economics. Provided by : Wikipedia. Located at : en.Wikipedia.org/wiki/Natural...urce_economics . License : CC BY-SA: Attribution-ShareAlike
- Natural resources. Provided by : Wikipedia. Located at : en.Wikipedia.org/wiki/Natural_resources . License : CC BY-SA: Attribution-ShareAlike
- Clean Air Act (United States). Provided by : Wikipedia. Located at : en.Wikipedia.org/wiki/Clean_A...United_States) . License : CC BY-SA: Attribution-ShareAlike
- depletion. Provided by : Wiktionary. Located at : en.wiktionary.org/wiki/depletion . License : CC BY-SA: Attribution-ShareAlike
- natural resource. Provided by : Wiktionary. Located at : en.wiktionary.org/wiki/natural_resource . License : CC BY-SA: Attribution-ShareAlike
- Nested sustainability-v2. Provided by : Wikipedia. Located at : en.Wikipedia.org/wiki/File:Ne...ability-v2.gif . License : CC BY-SA: Attribution-ShareAlike
- Alternative Energies. Provided by : Wikipedia. Located at : en.Wikipedia.org/wiki/File:Al...e_Energies.jpg . License : CC BY-SA: Attribution-ShareAlike
- Natural resource. Provided by : Wikipedia. Located at : en.Wikipedia.org/wiki/Natural_resource . License : CC BY-SA: Attribution-ShareAlike
- sustainable. Provided by : Wiktionary. Located at : en.wiktionary.org/wiki/sustainable . License : CC BY-SA: Attribution-ShareAlike
- externality. Provided by : Wiktionary. Located at : en.wiktionary.org/wiki/externality . License : CC BY-SA: Attribution-ShareAlike
- Resource. Provided by : Wikipedia. Located at : en.Wikipedia.org/wiki/Resource . License : CC BY-SA: Attribution-ShareAlike
- Externalities. Provided by : Wikipedia. Located at : en.Wikipedia.org/wiki/Externalities . License : CC BY-SA: Attribution-ShareAlike
- Resource allocation. Provided by : Wikipedia. Located at : en.Wikipedia.org/wiki/Resource_allocation . License : CC BY-SA: Attribution-ShareAlike
- Diesel-smoke. Provided by : Wikipedia. Located at : en.Wikipedia.org/wiki/File:Diesel-smoke.jpg . License : Public Domain: No Known Copyright
Search the site
Links to social media channels

The Sustainable Use of Natural Resources: The Governance Challenge
Still Only One Earth: Lessons from 50 years of UN sustainable development policy
Over-exploitation of natural resources harms the health of ecosystems and the wellbeing of people. In the face of environmental crises and growing inequality, we need to act, including developing extended producer responsibility and supply chain legislation, guaranteeing green public procurement, supporting technical innovation to enhance resource circularity, and adopting decision-making processes that include and respect women, Indigenous Peoples, and local communities. ( Download PDF ) ( See all policy briefs ) ( Subscribe to ENB )
Natural resources are central to human wellbeing. We cannot live without the clean air we breathe, the plants we eat, or the water we drink. We need natural resources to put roofs over our heads and heat our homes. We need them to survive and to thrive.
The concept of natural resources refers to naturally occurring living and non-living elements of the Earth system, including plants, fish, and fungi, but also water, soil, and minerals. A prominent way to think about natural resources is to look at them in terms of depletion risk: do they regenerate, and, if so, at what pace? Some resources, such as trees and plants, are renewable because they regenerate relatively quickly. Others, such as copper and oil, take much longer to form and are considered non-renewable. Together, natural resources make up a dense web of interdependence, forming ecosystems that also include humans. As such, the distribution of resources shapes the face of our planet and the local distinctiveness of our environments. People have formed different types of cultural, spiritual, and subsistence-based relationships with the natural environment, adopting value-systems that go beyond economic framings.
Nature makes human development possible but our relentless demand for the earth’s resources is accelerating extinction rates and devastating the world’s ecosystems. Joyce Msuya , Deputy Executive Director, United Nations Environment Programme
The use of natural resources has long been considered an element of both human rights and economic development, leading the United Nations, amid its work on advancing decolonization in the 1960s, to declare that “[t]he right of peoples and nations to permanent sovereignty over their natural wealth and resources must be exercised in the interest of their national development and of the well-being of the people of the State concerned” ( UN General Assembly Resolution 1803 (XVII) ).
Natural resources are often viewed as key assets driving development and wealth creation. Over time and with progressive industrialization, resource use increased. In some cases, exploitation levels came to exceed resources’ natural regeneration rates. Such overexploitation ultimately threatens the livelihoods and wellbeing of people who depend on these resources, and jeopardizes the health of ecosystems. This risk of resource depletion, notably manifesting in the form of fishery collapses, demonstrates the need to regulate natural resource use to better preserve resources and their ecosystems. The very first UN conference on environmental issues, the 1972 UN Conference on the Human Environment held in Stockholm, Sweden, adopted fundamental principles in this regard.
Stockholm Declaration
- Principle 2: “The natural resources of the earth, including the air, water, land, flora and fauna and especially representative samples of natural ecosystems, must be safeguarded for the benefit of present and future generations through careful planning or management, as appropriate.”
- Principle 3: “The capacity of the earth to produce vital renewable resources must be maintained and, wherever practicable, restored or improved.”
- Principle 5: “The non-renewable resources of the earth must be employed in such a way as to guard against the danger of their future exhaustion and to ensure that benefits from such employment are shared by all mankind.”
The Stockholm Declaration not only addressed resource depletion, but also benefit sharing: the objective to ensure that natural resource use not only benefits the few, but the many, both within and across countries. It also speaks to the principle of inter-generational equity: ensuring that today’s resource use does not compromise the availability of natural resources for future generations. In fact, natural resource use relates to all three dimensions of sustainability: social justice, environmental health, and economic development. The sustainable use of natural resources strives for balance between these dimensions: maintaining the long-term use of resources while maximizing social benefits and minimizing environmental impacts.
Natural Resource Use Has More than Tripled since 1970
Although the 1972 Stockholm Declaration laid out the fundamental principles for sustainable resource governance, the state of play half a century later is sobering. The International Resource Panel (IRP), launched by the United Nations Environment Programme (UNEP), found that the global average of material demand per capita grew from 7.4 tons in 1970 to 12.2 tons in 2017, with significant adverse impacts on the environment, notably increased greenhouse gas emissions.
The IRP also showed that “the use of natural resources and the related benefits and environmental impacts are unevenly distributed across countries and regions” (IRP, 2019, p. 27). For one, the per capita material footprint in high-income countries is thirteen times more than in low-income countries: 27 tons and 2 tons per capita, respectively. As WWF notes , “If everyone lived like an average resident of the USA, a total of four Earths would be required to regenerate humanity’s annual demand on nature.” What’s more, since they generally rely on resource extraction in other countries, high income countries outsource part of the environmental and social impacts of their consumption. At the same time, the IRP has reported that “the value created through these traded materials in the countries of origin is relatively low” (IRP, 2019, p. 65). This imbalance highlights the global discrepancies in the distribution of benefits and negative impacts stemming from resource use, with countries “rich” in valuable resources not always benefitting from their extraction, distribution, and use, yet suffering the most environmental harm.
Human actions threaten more species with global extinction now than ever before. Intergovernmental Science-Policy Platform on Biodiversity and Ecosystem Services 2019 Global Assessment Report on Biodiversity and Ecosystem Services
Fostering Sustainable Resource Governance
A vast array of norms, institutions, and actors influence decisions on natural resources, which is why we speak of natural resource governance. A plethora of national legislation, intergovernmental agreements, regional organizations, certification mechanisms, corporate codes of conduct, and multi-stakeholder partnerships create a complex web of rules affecting how natural resources are used and benefits thereof are distributed.

Since Stockholm, numerous multilateral agreements have developed a range of operational guidelines, targets, and standards. Some intergovernmental frameworks, such as the Convention on Biological Diversity (CBD) are broad in focus, while others are resource-specific ( Minamata Convention on Mercury ) or relate to a specific geographical area ( Convention on the Conservation of Antarctic Marine Living Resources ). Industry initiatives and multi-stakeholder partnerships often focus on specific resources or sectors. Examples of such initiatives include the Forest Stewardship Council , the Roundtable on Sustainable Palm Oil , the Extractive Industries Transparency Initiative , and the Better Cotton Initiative .
Citizens also have agency over natural resource use: through the representatives we elect to government, our activist engagement, and our consumption and transport choices. For instance, carefully considering food production cycles—what we eat, where and how it is grown, and how it arrives on our plate—can go towards addressing the impact that agricultural expansion has on forests, wetlands, and grassland ecosystems (FAO, 2018; IPBES, 2019). However, this needs to be coupled with systemic change across governance structures.
These mechanisms and institutions are not always complementary; in fact, at times they stand in conflict with one another. Consider, for instance, an energy corporation invoking the Energy Charter Treaty to file arbitration claims against a country’s decision to phase-out coal—a decision taken in accordance with its obligations under the Paris Agreement on Climate Change .
Balancing Rights and Interests over Natural Resources
Determining how people can—and should— access, benefit from, participate in decision-making on, and have responsibility over natural resources has been shaped by concepts such as property and rights .
On the one hand, property rights divide lands and territories into: private property, where rights are held by individuals or companies; common property, where rights are shared by a community; public property, where rights are held by government; and open access areas, where no specific rights are assigned (Aggrawal & Elbow, 2006). Property rights are closely tied to rights over natural resources, which include the right to use a resource, such as hunting in a forest; or management rights that grant authority to decide on use, for example imposing seasonal hunting restrictions. In terms of governance, different types of ownership and access rights can be held simultaneously by several actors: a wetland can be owned by the state, managed by a local council, and used as fishing grounds by communities.
The notion of tenure security indicates that an individual’s rights over natural resources and specific lands are recognized and enforceable. These rights are key to avoiding conflict and fostering social security as well as long-term sustainable resource use.
On the other hand, there are individual and collective rights regarding quality of life. The United Nations Declaration on the Rights of Peasants and Other People Working in Rural Areas ( UNDROP ), for example, stipulates that “[p]easants and other people working in rural areas have the right to have access to and to use in a sustainable manner the natural resources present in their communities that are required to enjoy adequate living conditions” and that they “have the right to participate in the management of these resources” (Article 5). UNDROP highlights the importance of small-scale sustainable practices, and the need to strengthen the protection and recognition of groups who have experienced historical marginalization and violent conflict over resource use.
Similarly, the UN Declaration on the Rights of Indigenous Peoples ( UNDRIP ) and International Labour Organization (ILO) Convention 169 ( ILO 169 ) protect the individual and collective rights of Indigenous Peoples. UNDRIP Article 8(2b) stipulates that states shall prevent and provide redress for “any action which has the aim or effect of dispossessing them of their lands, territories or resources.” Both texts also speak to the importance of ensuring the free, prior, and informed consent (FPIC) of Indigenous Peoples in relation to the use of their lands, with UNDRIP Articles 11(2) and 28 underscoring Indigenous Peoples’ right to redress for past FPIC infringements.
There is also the right to a healthy environment, enshrined in regional treaties, including procedural rights on access to information and decision-making processes, as well as the right to clean air, a safe climate, healthy food, safe water, a safe environment for work and play, and healthy ecosystems (UN Human Rights Council, 2019). Ultimately, the effectiveness of these advances in international law depends upon national governments’ readiness to implement them. To date, only 23 countries have ratified ILO 169, and many countries around the world have yet to adopt appropriate legislation to protect the rights enshrined in UNDRIP. To do so, and to protect associated rights under UNDROP and the right to a healthy environment, governments must adopt robust reforms across national policies, laws, programmes, and institutions that prompt shifts in country priorities and ensure the mainstreaming of environmental and social concerns across sectors, focusing especially on empowering marginalized groups. To ensure that decisions across society better address ecological and social wellbeing, prominent actors, including the UN Special Rapporteur on Human Rights and the Environment, are calling for human rights-based approaches to natural resource governance.
Overall, this constitutes a complex architecture, one that is dynamic in nature, often builds on customary practices, and requires balancing “competing” rights and interests through law and policy. Structures are seldom straightforward: there are often overlapping or even conflicting systems in place, and this influences the sustainability of resource governance.
States play a central role in balancing rights and interests. Regulations addressing the extractive sector determine how a corporation’s exclusive user rights may impact the general population’s right to a safe and healthy environment. Approaches to this balancing act, and the distribution, recognition, and safeguarding of rights, and the implementation of associated responsibilities, vary across states and change over time.
At times, this balance of interests favors more powerful actors. Stemming from historical legacies and trajectories in decision-making, structural inequalities exist across resource access, ownership, and tenure security (Oxfam, 2014). These issues disproportionately impact women , rural communities, and Indigenous Peoples, who are often cast as passive recipients to policy change, as opposed to rights holders and key actors in the sustainable management of natural resources.
Women have faced historical exclusion from decision-making processes related to land and resources (UN Women, 2020). Due to enduring patriarchal gender norms across the world, they hold less control than men over the lands and resources they traditionally use and rely on for their livelihoods and wellbeing. Based on an analysis of 180 countries, the Organisation for Economic Co-operation and Development (OECD) found that out of the 164 countries that explicitly recognize women’s rights to own, use, and make decisions regarding land on par with men, only 52 countries guarantee these rights in both law and practice (OECD, 2019). As such, it is important that states ensure that women’s rights over natural resources are realized and protected through appropriate mechanisms.

Indigenous Peoples also struggle to have their rights recognized. For instance, in Finland, Sweden, and Canada, legal disputes have arisen over the challenge of balancing between states’ sovereign right to govern and exploit natural resources, and Indigenous Peoples’ rights to self-determination over traditional territories and customary resource use. Globally, conflicts have also emerged over specific policy approaches, such as conservation methods relying on models of strictly protected areas, or the expansion of large infrastructure, such as the installation of hydraulic dams, which contribute to the displacement of Indigenous and rural peoples.
The expansion of international investment treaties further aggravates existing power differentials. In fostering the commercialization and privatization of land and resources, and by often prioritizing investors’ rights and interests over those held by local peoples, they risk restricting public-interest policies and undermine the public’s access to remedial action (Cotula, 2015, 2016).
The Need for Inclusive Governance
Activists and practitioners working to safeguard rights linked to natural resources and secure tenure have been lobbying for strengthened empowerment and participation of local groups, arguing that this fosters more sustainable and equitable resource governance. Alliances between women, youth, Indigenous Peoples, and local community groups have emerged, connecting local-to-global efforts, and bringing international attention to injustices. This includes grassroots alliances such as La Vía Campesina , which has lobbied to protect farmers’ and peasants’ rights since the 1990s and was instrumental in the creation and adoption of UNDROP.
Inclusive decision making is key for sustainable resource governance. Just as gender norms have influenced structures for access and use, they have also shaped our behaviors and the knowledge we acquire, with women holding unique agroecological expertise linked to crop resilience and nutrition (UN Women, 2018). So, unless decision-making processes are gender-responsive and inclusive, they risk overlooking women’s specific needs and roles, and will fail to ensure the inclusion of ecological knowledge important for enabling sustainable practices.
The same can be said for including Indigenous Peoples and local communities in resource governance. The second edition of the CBD’s Local Biodiversity Outlooks illustrates their significant contributions to the safeguarding and sustainable use of natural resources and biodiversity. Important benefits come with inclusive and community-led governance structures and decision-making processes, which, in addition to protecting and enabling sustainable use of resources, can strengthen community support systems and local economies, as well as revitalize Indigenous and local knowledges and languages.
The Need for Transformative Change
Despite efforts since the 1970s, current trends in natural resource use are unsustainable, with potentially devastating results. The 2019 IPBES Global Assessment Report underscored that transformative change is necessary to protect the resources upon which human life and wellbeing depends. The Report also acknowledges that, by its very nature, transformative change is often opposed by those with interests vested in the status quo. Civil society actors therefore underscore the importance for governments to address vested interests and foster inclusive decision making, along with a re-balancing of priorities with regards to rights and interests in order to ensure ecological integrity and social justice (Allan, et.al., 2019). The Local Biodiversity Outlooks mentioned earlier offer important examples of bottom-up approaches to resource governance that can foster sustainability while also addressing historical inequalities.
Bearing in mind global and local inequalities in the distribution of resource use and benefits, achieving transformative change requires bold governmental action, both domestically and in international fora. We need fundamental shifts in production and consumptions patterns, careful attention to value and supply chains, and the fostering of circular resource use and circular economies. Resource circularity breaks with the linear model of “extract-use-discard” towards a “waste-as-a-resource” model that fosters a reduced need for resource extraction, as well as encourages increased reuse, repair and recycling. These objectives are already enshrined in the 2030 Agenda for Sustainable Development , with governments aiming to achieve the sustainable management and efficient use of natural resources by 2030 . While implementation has been too slow (IPBES, 2019), there is increased attention to fostering resource circularity, hand in hand with efforts to promote secure labor standards and reduce environmental impacts of resource exploitation. Most notable in this regard are legislative initiatives that increase producers’ responsibility for the impacts of their products throughout their lifecycle. Placing responsibility for post-use disposal on manufacturers significantly increases the material recovery rate and incentivizes less wasteful product design (OECD, 2016).

To better balance the three dimensions of sustainable resources governance—social justice, environmental health, and economic development—we must rethink our economic, social, political, and technological systems that currently enable damaging production practices and wasteful resource consumption. Other ways of living are possible, from the ways we structure our societies and economies, the relationships we form with each other and with our ecosystems, to ensuring that the priorities of our leaders align with the interests of the many rather than the few. To realize these shifts, governments should develop extended producer responsibilities and supply chain legislation to enhance fairer distribution of benefits and harms stemming from resource use and promote the protection of human rights in ways that ensure ecological wellbeing and social justice.
Decision making must be inclusive and account for the needs, rights, and knowledges of historically marginalized communities and groups. Governance structures must recognize and support pre-existing sustainable practices at local and regional levels, as well as nourish the emergence of more sustainable patterns of resource use and management. This will require strengthening tenure rights and re-distributing power across all stages of decision-making.
Works Consulted
Aggarwal, S. & Elbow, K. (2016). The role of property rights in natural resource management, good governance and empowerment of the rural poor. USAID. https://www.land-links.org/wp-content/uploads/2016/09/USAID_Land_Tenure_Property_Rights_and_NRM_Report.pdf
Allan, J.I., Antonich, B., Bansard, J.S., Luomi, M., & Soubry, B. (2019). Summary of the Chile/Madrid Climate Change Conference: 2-15 December 2019. Earth Negotiations Bulletin , 12(775). https://enb.iisd.org/download/pdf/enb12775e.pdf
Cotula, L. (2015). Land rights and investment treaties. IIED. https://pubs.iied.org/sites/default/files/pdfs/migrate/12578IIED.pdf
Cotula, L. (2016). Rethinking investment treaties to advance human rights. IIED Briefing. https://pubs.iied.org/sites/default/files/pdfs/migrate/17376IIED.pdf
Food and Agriculture Organization of the United Nations. (2018). Sustainable food systems: Concept and framework. http://www.fao.org/3/ca2079en/CA2079EN.pdf
Forest Peoples Programme, International Indigenous Forum on Biodiversity, Indigenous Women’s Biodiversity Network, Centres of Distinction on Indigenous and Local Knowledge, & Secretariat of the Convention on Biological Diversity. (2020). Local biodiversity outlooks 2 . https://www.cbd.int/gbo/gbo5/publication/lbo-2-en.pdf
Intergovernmental Science-Policy Platform on Biodiversity and Ecosystem Services. (2019). Global assessment report on biodiversity and ecosystem services. https://www.ipbes.net/global-assessment
International Resource Panel. (2019). Global resources outlook 2019: Natural resources for the future we want. UN Environment Programme. https://www.resourcepanel.org/reports/global-resources-outlook
Organisation for Economic Co-operation and Development. (2016). Extended producer responsibility: Updated guidance for efficient waste management. https://doi.org/10.1787/9789264256385-en
Organisation for Economic Co-operation and Development. (2019). Social institutions and gender index 2019 global report: Transforming challenges into opportunities. https://doi.org/10.1787/bc56d212-en
Oxfam. (2014). Even it up: Time to end extreme inequality. https://www-cdn.oxfam.org/s3fs-public/file_attachments/cr-even-it-up-extreme-inequality-291014-en.pdf
UN Human Rights Council. (2019). Report by the Special Rapporteur on the issue of human rights obligations relating to the enjoyment of a safe, clean, healthy and sustainable environment. A/HRC/43/53. https://undocs.org/A/HRC/43/53
UN Women (2018). Towards a gender-responsive implementation of the Convention on Biological Diversity. https://www.unwomen.org/en/digital-library/publications/2018/11/towards-a-gender-responsive-implementation-of-the-convention-on-biological-diversity
UN Women (2020). Realizing women’s rights to land and other productive resources. 2nd ed. https://www.unwomen.org/en/digital-library/publications/2020/10/realizing-womens-rights-to-land-and-other-productive-resources-2nd-edition
Additional downloads
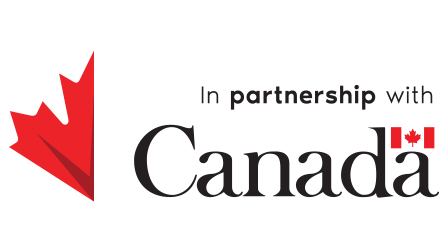
Government of Canada, Global Affairs Canada
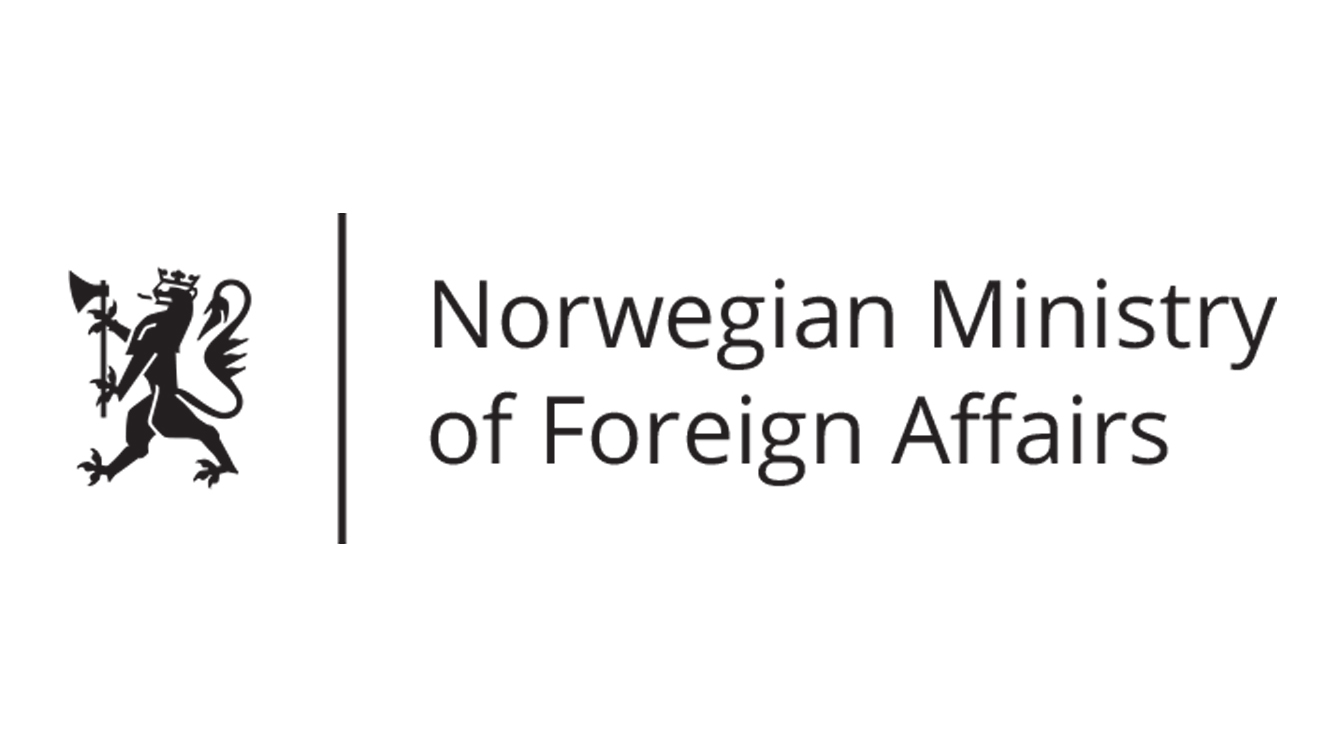
Government of Norway, Ministry of Foreign Affairs

Government of Sweden, Ministry of Environment
Deep dive details, you might also be interested in, igf case study: leveraging technologies for gender equality in mining communities.
How can sharing technological infrastructure support gender equality and serve the broad betterment of mining communities?
February 2, 2024
How to Regulate Our Waste-Full World
Going forward, the legitimacy of global governance of hazardous wastes may rest on its ability to enable governments protect the most vulnerable.
July 26, 2021
ASGM Tailings Management and Reprocessing Governance
This report outlines technical aspects, governance frameworks, and policy recommendations for artisanal and small-scale gold mining (ASGM) tailings management and reprocessing.
May 6, 2024
Financial Benefit-Sharing Issues for Critical Minerals: Challenges and opportunities for producing countries
Exploring nuances in the key features of critical minerals and the new challenges and opportunities they present to fiscal regulation.
March 22, 2024
Our resources are running out. These charts show how urgently action is needed
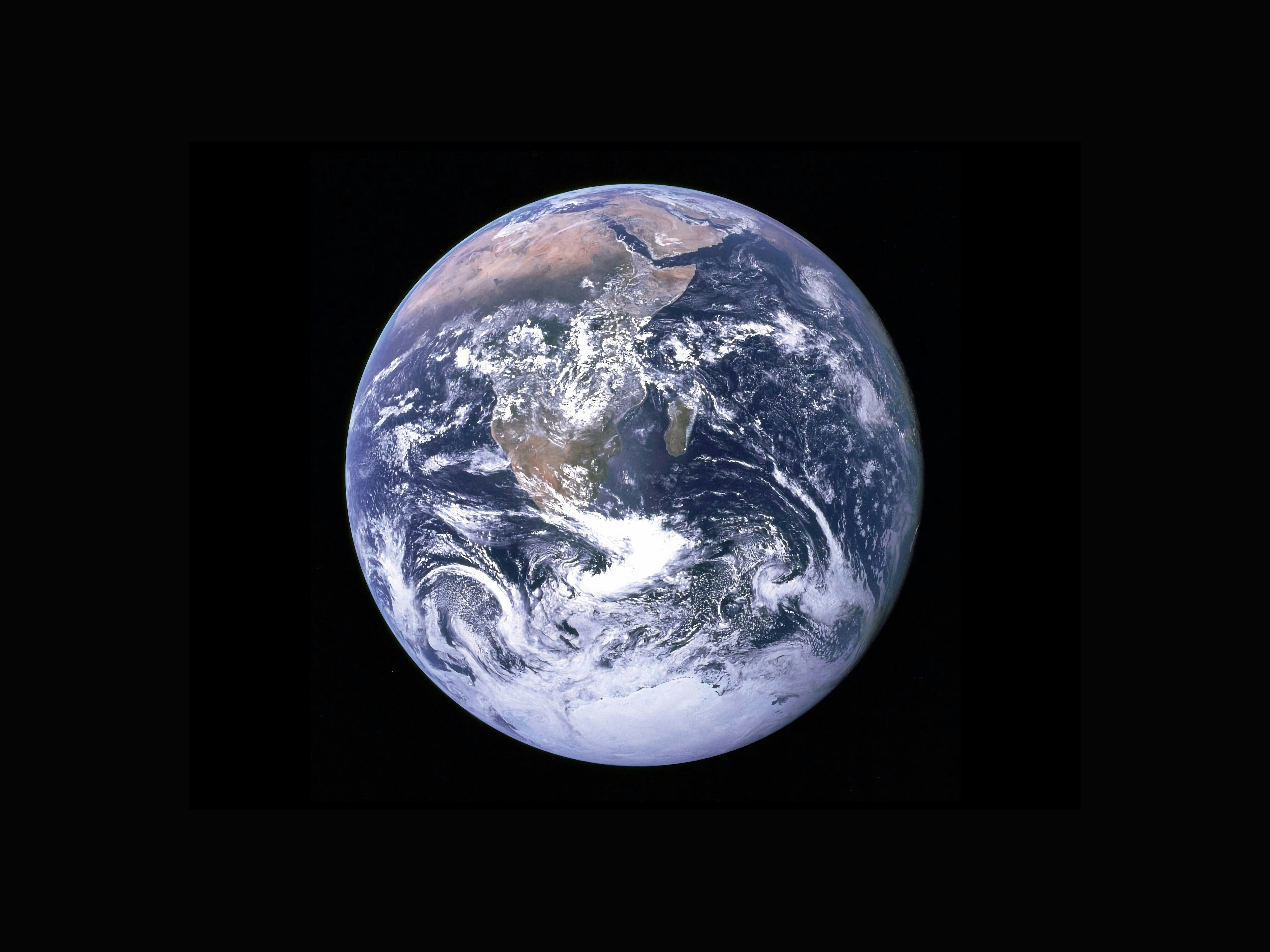
“With decisive action by politicians and the private sector, a decent life for all is possible without costing the Earth," says the IRP’s Co-Chair, Janez Potočnik. Image: Unsplash/NASA
.chakra .wef-1c7l3mo{-webkit-transition:all 0.15s ease-out;transition:all 0.15s ease-out;cursor:pointer;-webkit-text-decoration:none;text-decoration:none;outline:none;color:inherit;}.chakra .wef-1c7l3mo:hover,.chakra .wef-1c7l3mo[data-hover]{-webkit-text-decoration:underline;text-decoration:underline;}.chakra .wef-1c7l3mo:focus,.chakra .wef-1c7l3mo[data-focus]{box-shadow:0 0 0 3px rgba(168,203,251,0.5);} Emma Charlton
.chakra .wef-9dduvl{margin-top:16px;margin-bottom:16px;line-height:1.388;font-size:1.25rem;}@media screen and (min-width:56.5rem){.chakra .wef-9dduvl{font-size:1.125rem;}} Explore and monitor how .chakra .wef-15eoq1r{margin-top:16px;margin-bottom:16px;line-height:1.388;font-size:1.25rem;color:#F7DB5E;}@media screen and (min-width:56.5rem){.chakra .wef-15eoq1r{font-size:1.125rem;}} Future of the Environment is affecting economies, industries and global issues
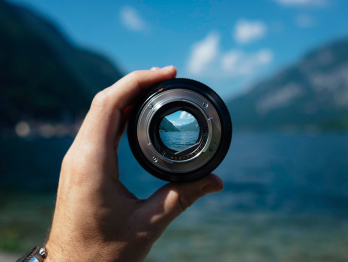
.chakra .wef-1nk5u5d{margin-top:16px;margin-bottom:16px;line-height:1.388;color:#2846F8;font-size:1.25rem;}@media screen and (min-width:56.5rem){.chakra .wef-1nk5u5d{font-size:1.125rem;}} Get involved with our crowdsourced digital platform to deliver impact at scale
Stay up to date:, future of the environment.
- Global natural resource consumption is forecast to rise 60% by 2060, compared with 2020 levels, according to the United Nations.
- Increasing demand for resources is due to urbanization, industrialization and a growing population, which is leading to severe consequences such as biodiversity loss, water stress, climate change and air pollution, it says.
- Disrupted supply chains for critical goods and resources were among the top risks identified in the World Economic Forum’s Global Risks Report 2024 .
We’re racing into a resources crisis that’s set to intensify unless urgent action is taken.
Global natural resource consumption is predicted to increase by 60% by 2060, compared with 2020 levels , according to the United Nations Environment Programme's Global Resources Outlook, a report by the UN's International Resource Panel. That’s after material use grew more than three times over the past 50 years, it said.
Resources include crops for food, wood for energy, fossil fuels, metals like iron, aluminium and copper, non-metallic minerals, as well as land and water.
“It is no longer whether a transformation towards global sustainable resource consumption and production is necessary, but how to urgently make it happen,” said Janez Potocnik and Izabella Teixeirq, International Resource Panel (IRP) co-chairs.
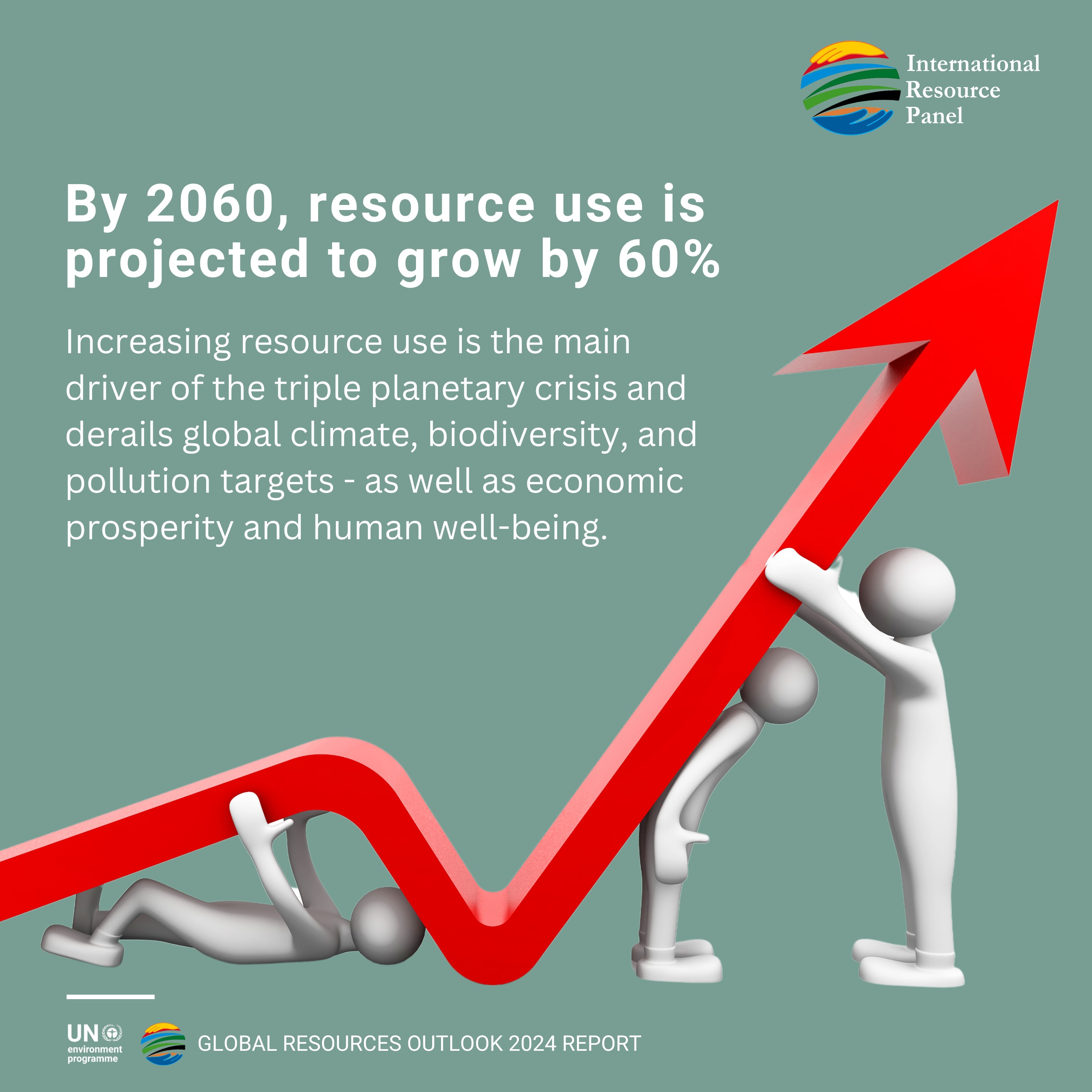
The pace at which we’re using our planet’s resources has a connection to almost every aspect of our lives. This exploitation is the main driver for the triple planetary crises , which are defined by the United Nations as the climate crisis, biodiversity loss and the pollution crisis, according to the IRP.
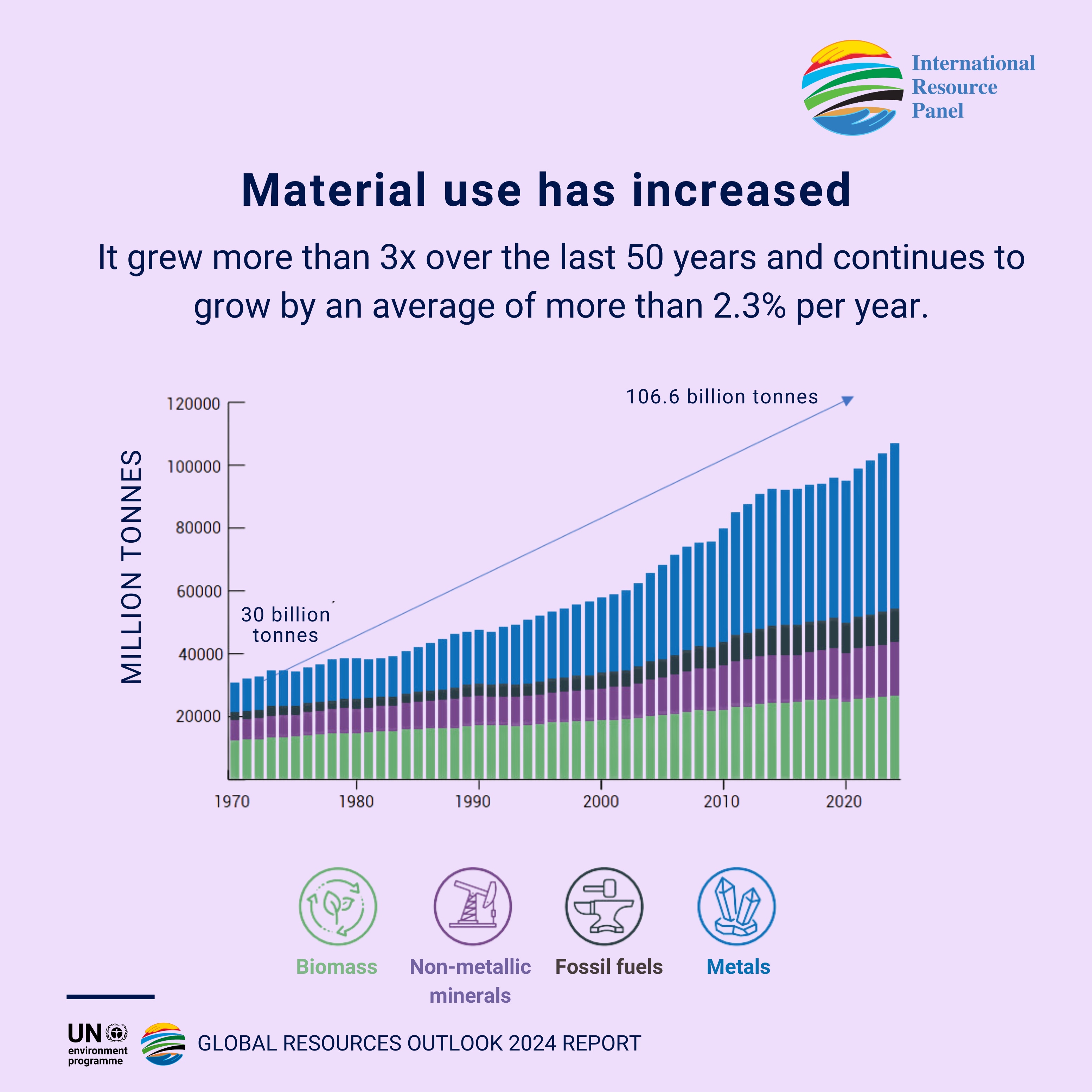
The Global Rewiring report highlighted that collective thinking on global value chains is changing due to increasing disruptions driven by geopolitical tension, climate change, and technological shifts.
The World Economic Forum’s Centre for Advanced Manufacturing and Supply Chains is at the forefront of future-proofing the global manufacturing industry. It supports sustainable growth by fostering innovation and accelerating the adoption of inclusive technology.
Learn more about our impact:
- The Global Lighthouse Network : We have brought together 132 manufacturing factories from various industries who are applying advanced technologies to boost productivity, enabling them to scale and replicate innovations.
- Circular value chains : We actively support manufacturers in incubating new pilots that reinforce trust in circular value chains, such as authenticating fashion products in second-hand markets and exchanging CO2 footprint data across supply chains .
- Resiliency : In partnership with Kearney, we developed the Resiliency Compass to help guide companies in navigating supply chain disruptions and enhancing their resilience.
Want to know more about our centre’s impact or get involved? Contact us .
Disrupted supply chains for critical goods and resources were among the top risks identified in the World Economic Forum’s Global Risks Report 2024 for the current risk landscape. Environmental risks were another significant concern identified in that report, with extreme weather ranked as the top risk likely to cause a global crisis in 2024.
Even so, the writers of the report still see scope to reduce resource use while growing the economy and achieving the other UN Sustainable Development Goals (SDGs). This will require a “decoupling” so that the environmental impacts of resource use fall, while the well-being contributions increase, they say.
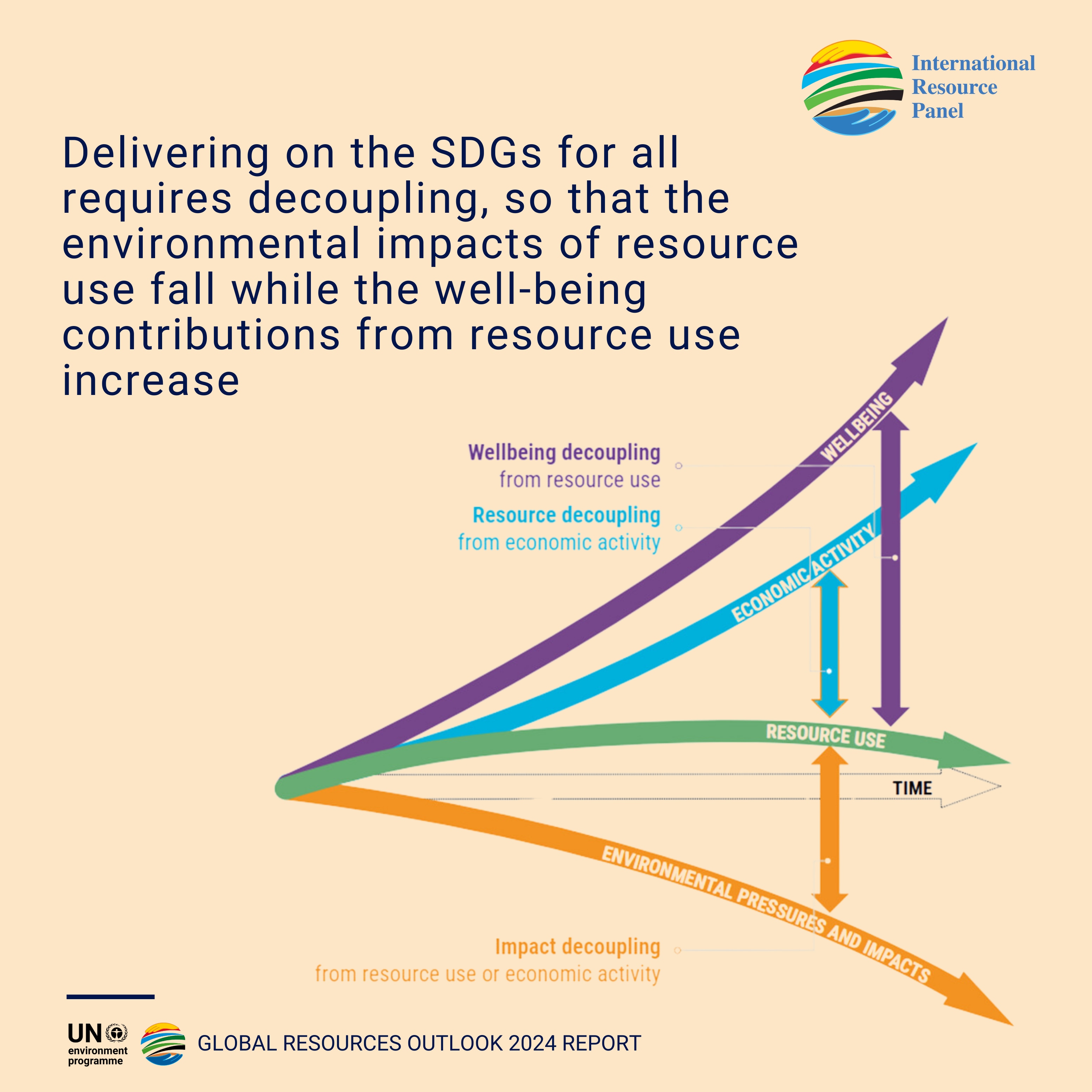
“Reorienting demand and allowing resource use to grow where it is most needed will open pathways to achieving the SDGs and a shared and equitable prosperity for all,” the report says. Bold policy action will be required and there must be a “much stronger focus on demand-side – consumption – measures”.
High-income countries use six times more materials per capita and are responsible for 10 times more climate impacts per capita than low-income countries. Material inequalities must be addressed as a core element of any approach, the report warns.
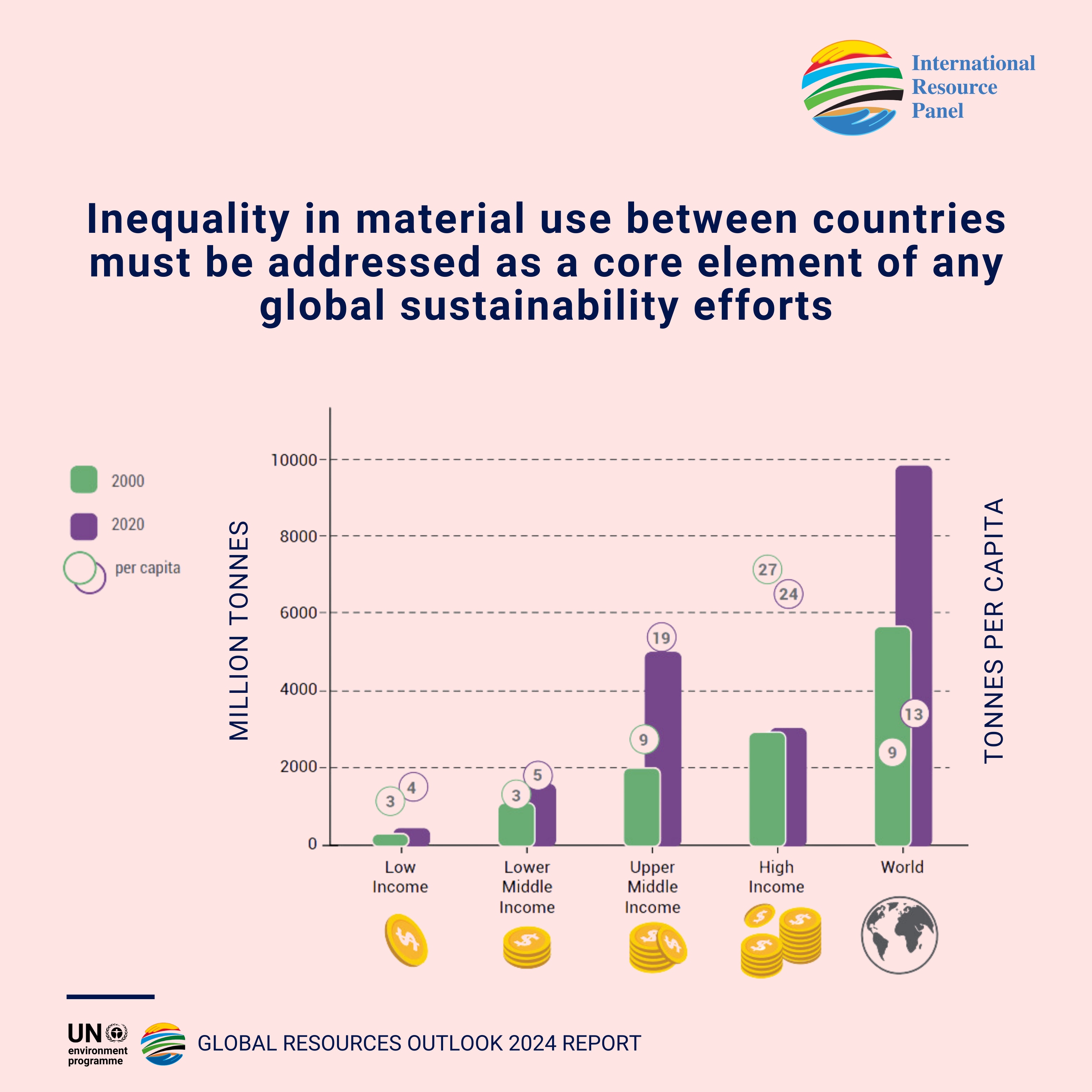
In areas where consumption levels are high, there needs to be a focus on lowering resource and material consumption levels and on efficiency. This could help reduce around 30% of resource use around the world compared with historical trends, it says. In countries where resource use needs to grow, it is possible to use strategies that maximize the value we get from resources.
Directing finance towards sustainable resource use is one potential solution, the report suggests, by making sure the true costs of resources are reflected in the structure of the economy. Providing consumers with information and access to sustainable goods and services is also crucial.
Regulation has an important role to play in disincentivizing or banning resource-intensive options – for example non-essential single-use plastic products, the report points out. Business models that build in refuse, reduce, eco-design, reuse, repair, and recycling initiatives are also necessary.
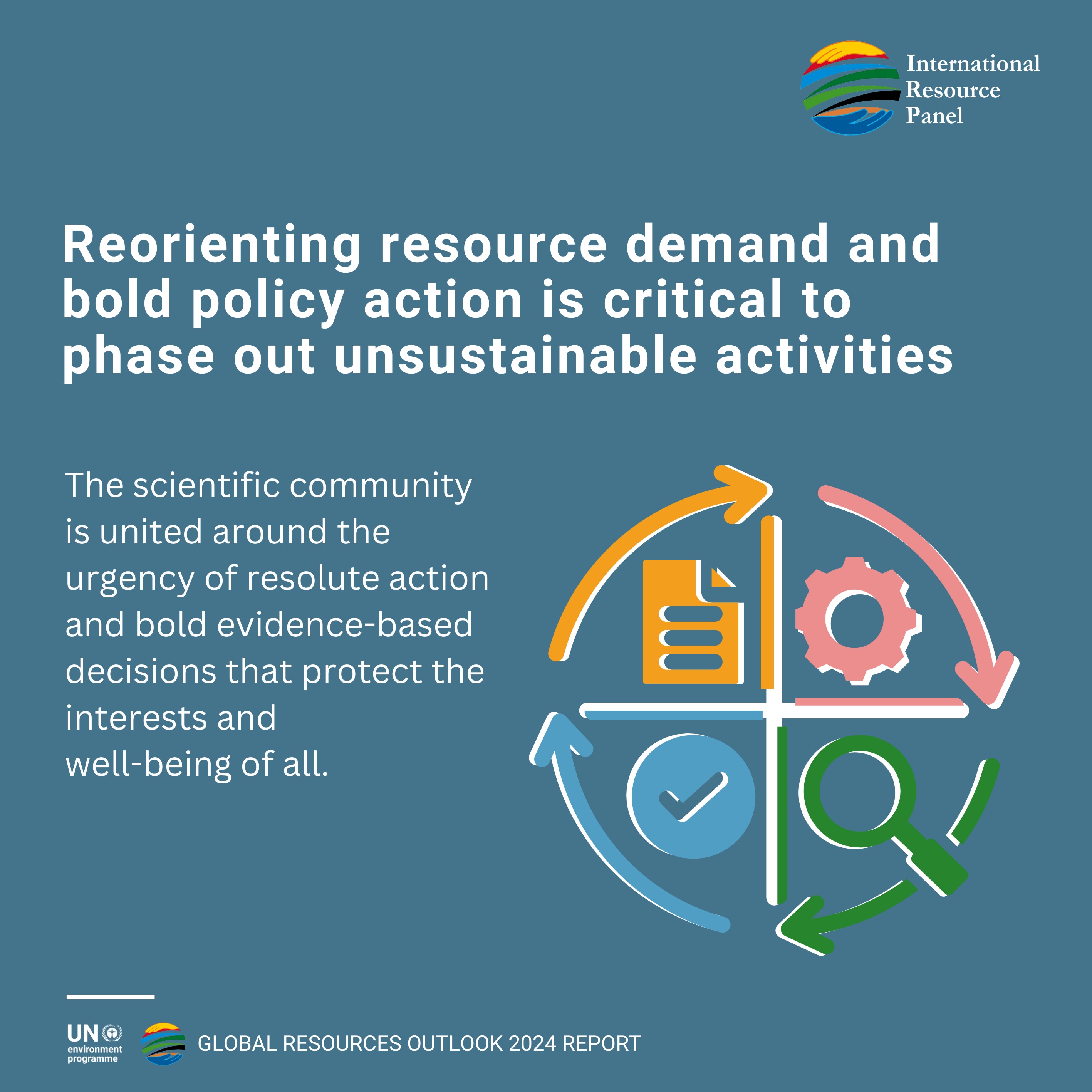
Much needs to be done to transform our built environments, and the ways we move around, and consume food and energy. High- and upper-middle-income countries could also shift away from animal protein.
“We should not accept that meeting human needs must be resource intensive, and we must stop stimulating extraction-based economic success,” said the IRP’s Co-Chair, Janez Potočnik. “With decisive action by politicians and the private sector, a decent life for all is possible without costing the Earth.”
Have you read?
Why we must optimize supply chains for the sharing economy, this explorer and conservationist is training citizen scientists to save the planet, these are the biggest global risks we face in 2024 and beyond, for manufacturers, the circular economy strengthens supply chains. here's how, don't miss any update on this topic.
Create a free account and access your personalized content collection with our latest publications and analyses.
License and Republishing
World Economic Forum articles may be republished in accordance with the Creative Commons Attribution-NonCommercial-NoDerivatives 4.0 International Public License, and in accordance with our Terms of Use.
The views expressed in this article are those of the author alone and not the World Economic Forum.
Related topics:
The agenda .chakra .wef-n7bacu{margin-top:16px;margin-bottom:16px;line-height:1.388;font-weight:400;} weekly.
A weekly update of the most important issues driving the global agenda
.chakra .wef-1dtnjt5{display:-webkit-box;display:-webkit-flex;display:-ms-flexbox;display:flex;-webkit-align-items:center;-webkit-box-align:center;-ms-flex-align:center;align-items:center;-webkit-flex-wrap:wrap;-ms-flex-wrap:wrap;flex-wrap:wrap;} More on Sustainable Development .chakra .wef-17xejub{-webkit-flex:1;-ms-flex:1;flex:1;justify-self:stretch;-webkit-align-self:stretch;-ms-flex-item-align:stretch;align-self:stretch;} .chakra .wef-nr1rr4{display:-webkit-inline-box;display:-webkit-inline-flex;display:-ms-inline-flexbox;display:inline-flex;white-space:normal;vertical-align:middle;text-transform:uppercase;font-size:0.75rem;border-radius:0.25rem;font-weight:700;-webkit-align-items:center;-webkit-box-align:center;-ms-flex-align:center;align-items:center;line-height:1.2;-webkit-letter-spacing:1.25px;-moz-letter-spacing:1.25px;-ms-letter-spacing:1.25px;letter-spacing:1.25px;background:none;padding:0px;color:#B3B3B3;-webkit-box-decoration-break:clone;box-decoration-break:clone;-webkit-box-decoration-break:clone;}@media screen and (min-width:37.5rem){.chakra .wef-nr1rr4{font-size:0.875rem;}}@media screen and (min-width:56.5rem){.chakra .wef-nr1rr4{font-size:1rem;}} See all
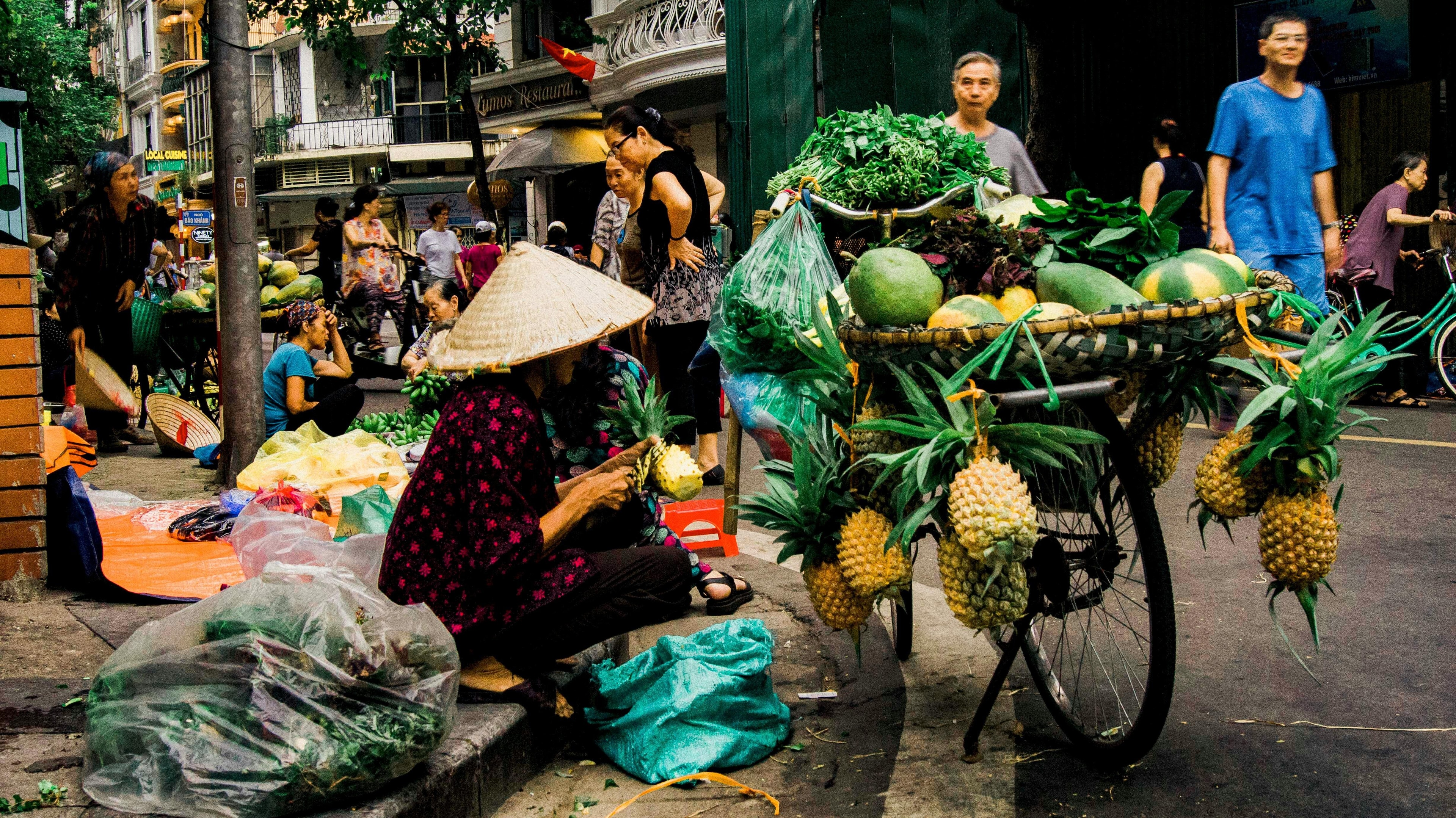
5 ways businesses can help to alleviate poverty
Sreevas Sahasranamam and Vivek Soundararajan
May 3, 2024
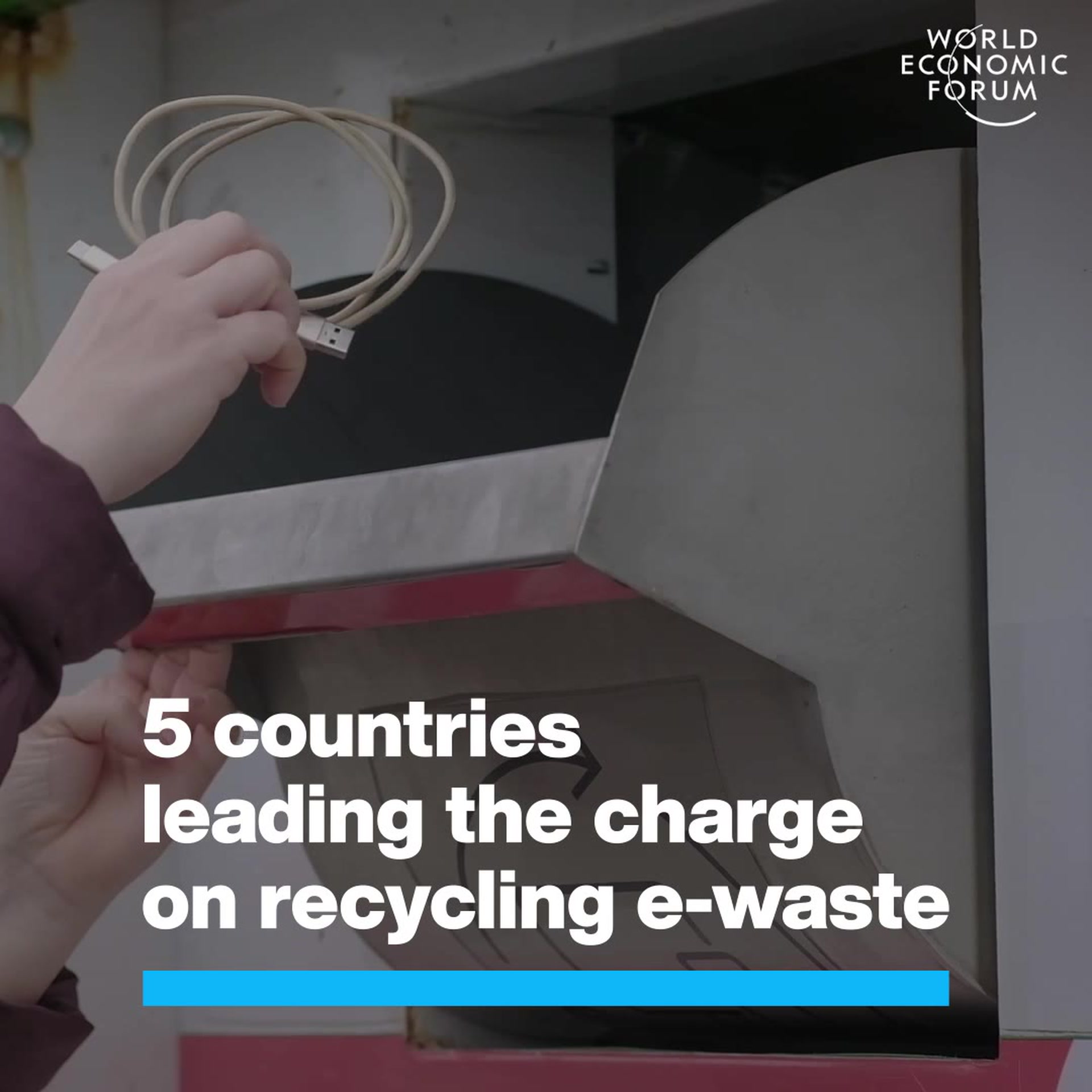
5 countries leading the charge on recycling e-waste
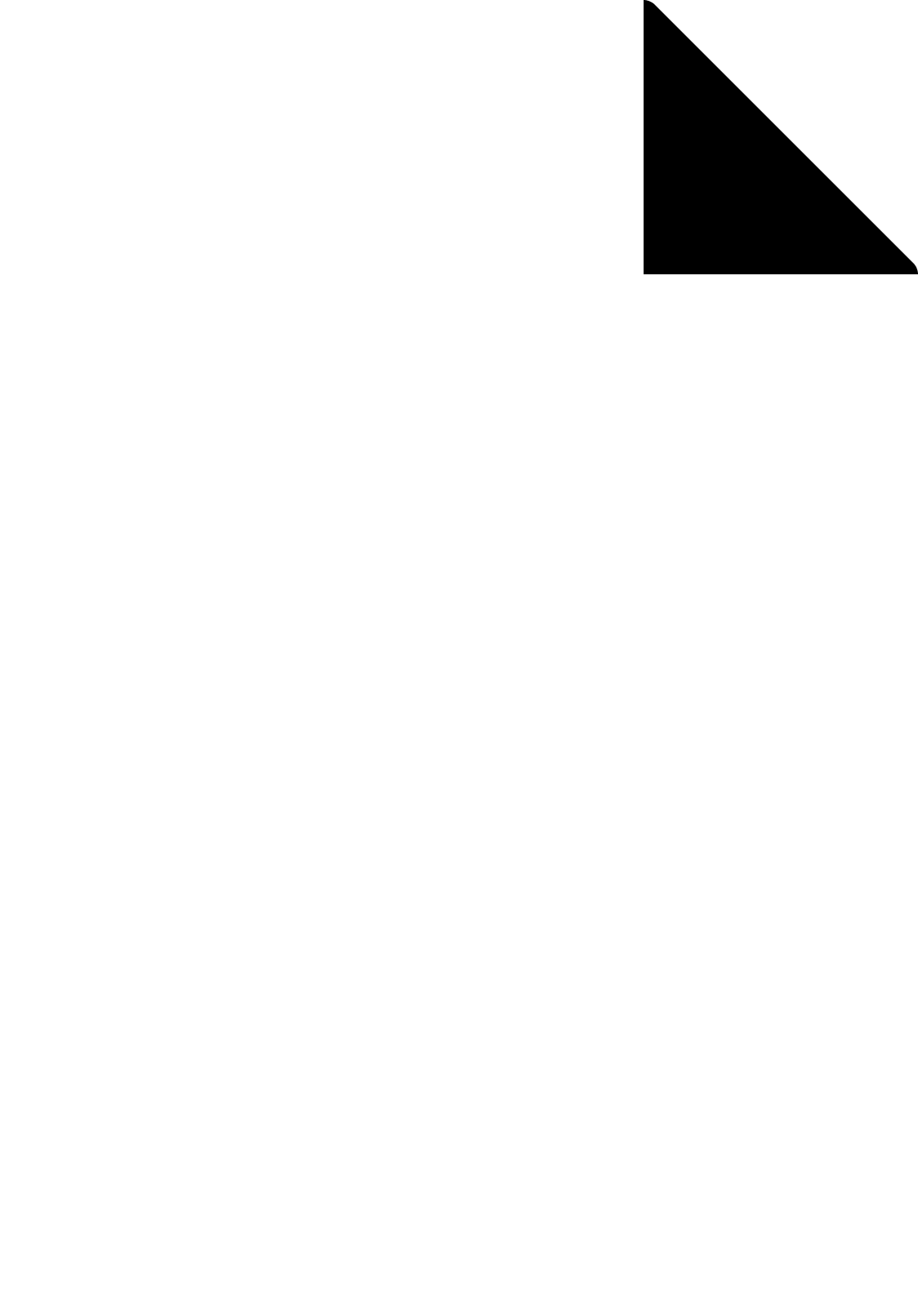
Reducing Embodied Carbon in Cities: Nine Solutions for Greener Buildings and Communities
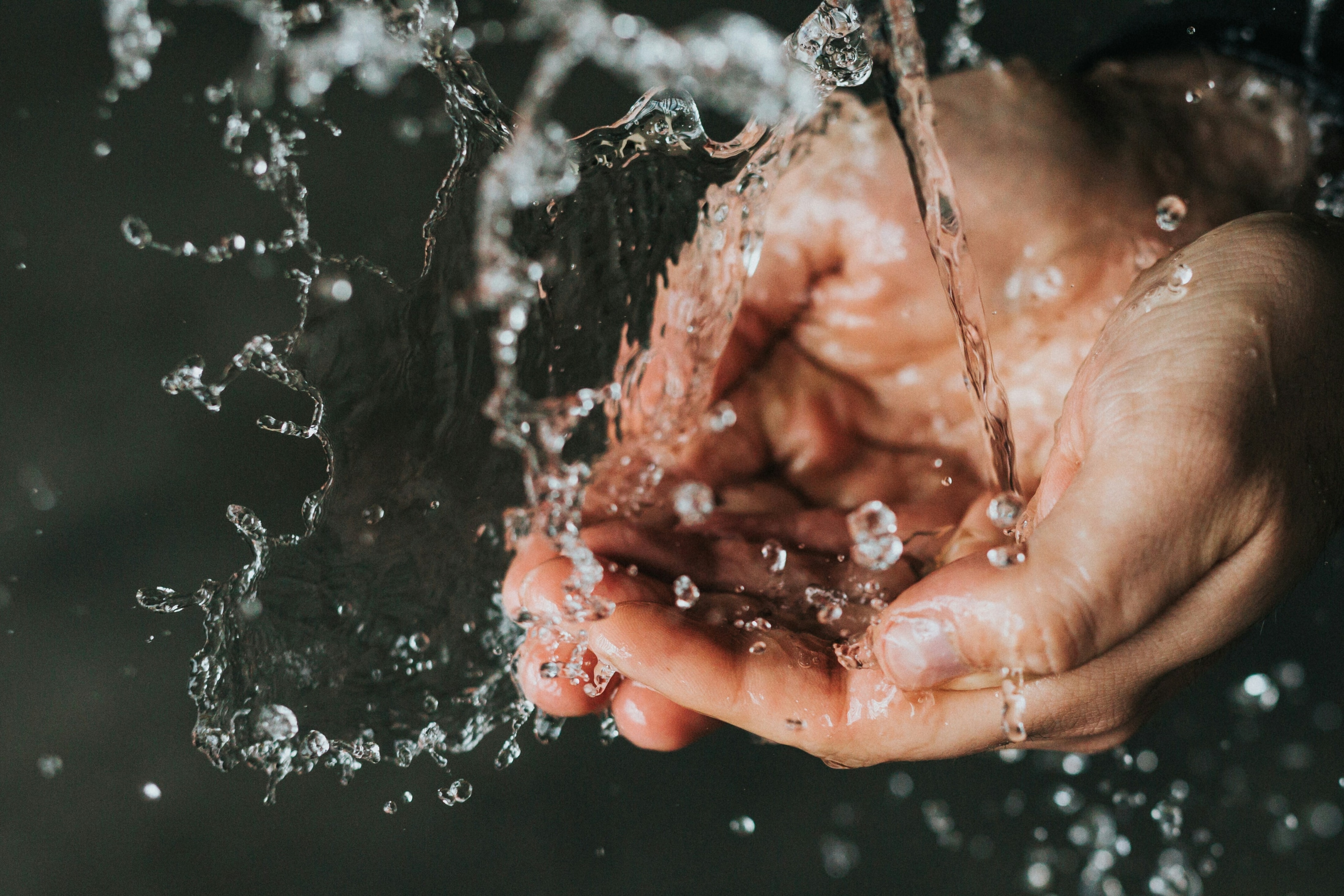
Desalination: What is it and how can it help tackle water scarcity?
Johnny Wood
April 15, 2024
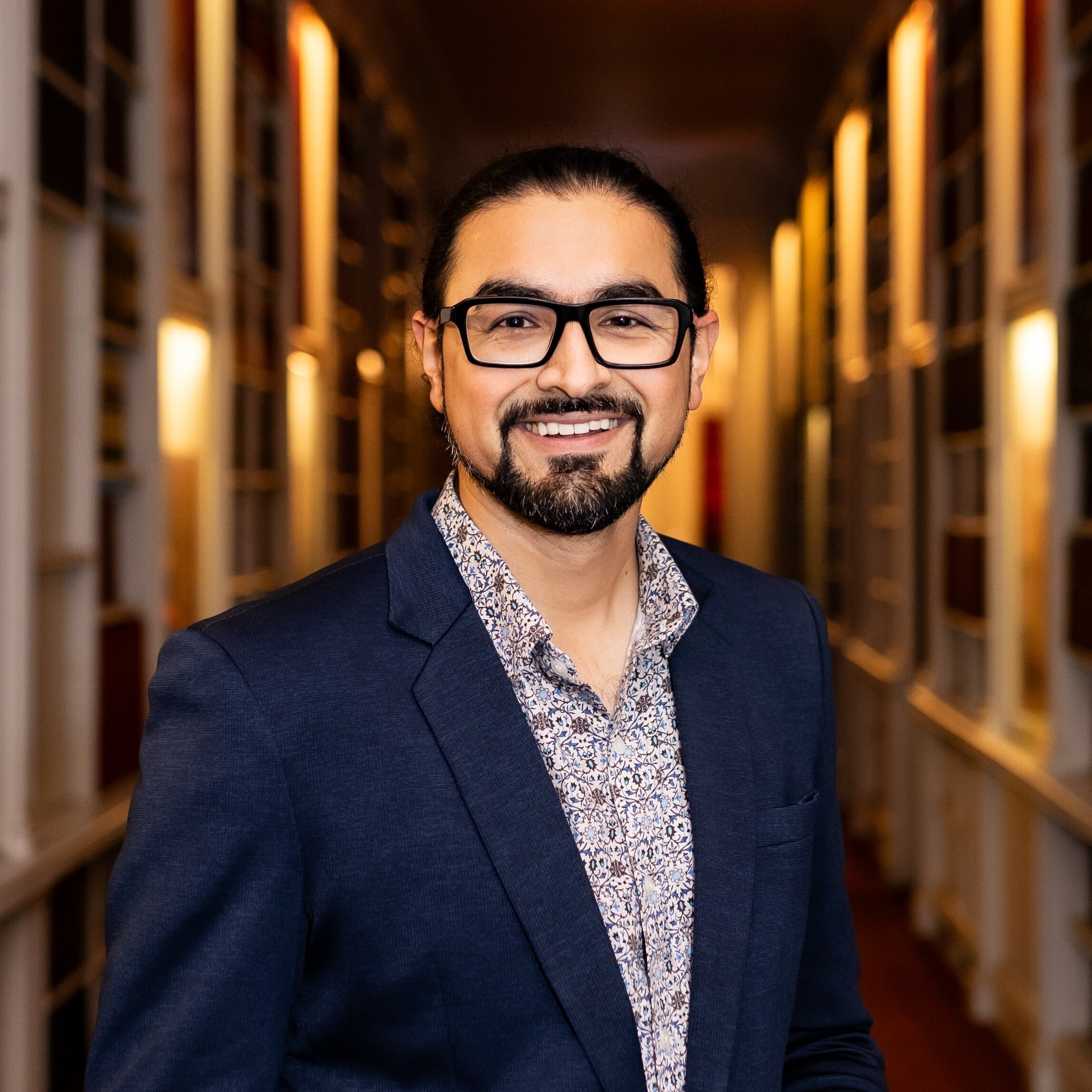
'It's now cheaper to save the world than destroy it': author Akshat Rathi on Climate Capitalism
Robin Pomeroy and Sophia Akram
April 10, 2024
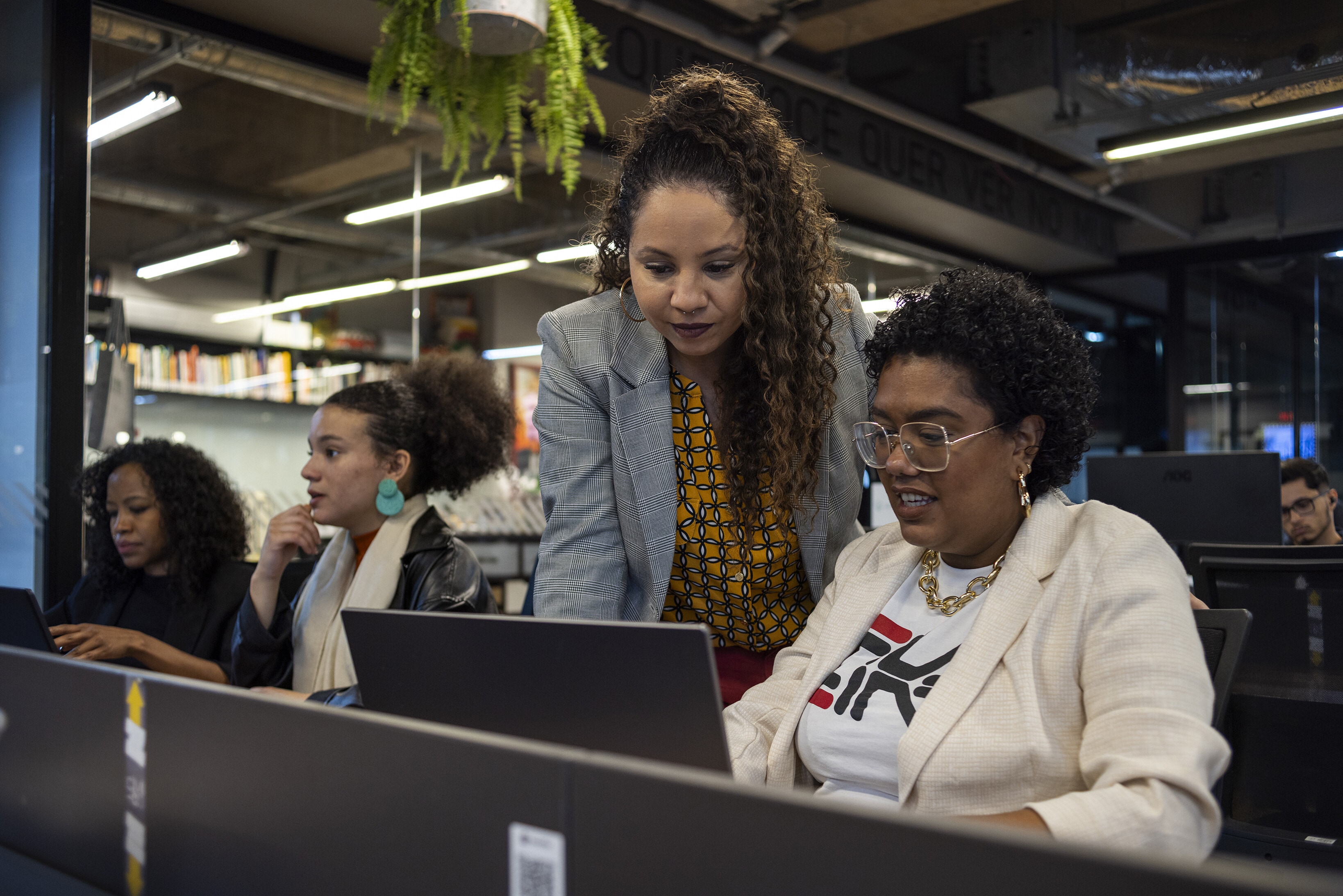
3 social economy innovators that are driving change in Brazil
Eliane Trindade
April 4, 2024
Module: Income Distribution
Reading: natural resources and conservation, natural resources and conservation.
Natural resources are the gifts of nature. They include everything from oil to fish in the sea to magnificent scenic vistas. The stock of a natural resource is the quantity of the resource with which the earth is endowed. For example, a certain amount of oil lies in the earth, a certain population of fish live in the sea, and a certain number of acres make up an area such as Yellowstone National Park or Manhattan. These stocks of natural resources, in turn, can be used to produce a flow of goods and services. Each year, we can extract a certain quantity of oil, harvest a certain quantity of fish, and enjoy a certain number of visits to Yellowstone.
As with capital, we examine the allocation of natural resources among alternative uses across time. By definition, natural resources cannot be produced. Our consumption of the services of natural resources in one period can affect their availability in future periods. We must thus consider the extent to which the expected demands of future generations should be taken into account when we allocate natural resources.
Natural resources often present problems of property rights in their allocation. A resource for which exclusive property rights have not been defined will be allocated as a common property resource. In such a case, we expect that the marketplace will not generate incentives to use the resource efficiently. In the absence of government intervention, natural resources that are common property may be destroyed. In this section, we shall consider natural resources for which exclusive property rights have been defined. The public sector’s role in the allocation of common property resources is investigated in the module on the environment.
We can distinguish two categories of natural resources, those that are renewable and those that are not. A renewable natural resource is one whose services can be used in one period without necessarily reducing the stock of the resource that will be available in subsequent periods. The fact that they can be used in such a manner does not mean that they will be; renewable natural resources can be depleted. Wilderness areas, land, and water are renewable natural resources. The consumption of the services of an exhaustible natural resource, on the other hand, necessarily reduces the stock of the resource. Oil and coal are exhaustible natural resources.
Exhaustible Natural Resources
Owners of exhaustible natural resources can be expected to take the interests of future as well as current consumers into account in their extraction decisions. The greater the expected future demand for an exhaustible natural resource, the greater will be the quantity preserved for future use.
Expectations and Resource Extraction
Suppose you are the exclusive owner of a deposit of oil in Wyoming. You know that any oil you pump from this deposit and sell cannot be replaced. You are aware that this is true of all the world’s oil; the consumption of oil inevitably reduces the stock of this resource.
If the quantity of oil in the earth is declining and the demand for this oil is increasing, then it is likely that the price of oil will rise in the future. Suppose you expect the price of oil to increase at an annual rate of 15%.
Given your expectation, should you pump some of your oil out of the ground and sell it? To answer that question, you need to know the interest rate. If the interest rate is 10%, then your best alternative is to leave your oil in the ground. With oil prices expected to rise 15% per year, the dollar value of your oil will increase faster if you leave it in the ground than if you pump it out, sell it, and purchase an interest-earning asset. If the market interest rate were greater than 15%, however, it would make sense to pump the oil and sell it now and use the revenue to purchase an interest-bearing asset. The return from the interest-earning asset, say 16%, would exceed the 15% rate at which you expect the value of your oil to increase. Higher interest rates thus reduce the willingness of resource owners to preserve these resources for future use.
Figure 13.8. Future Generations and Exhaustible Natural Resources. The current demand D for services of an exhaustible resource is given by the marginal revenue product (MRP). S1 reflects the current marginal cost of extracting the resource, the prevailing interest rate, and expectations of future demand for the resource. The level of current consumption is thus at Q1. If the interest rate rises, the supply curve shifts to S2, causing the price of the resource to fall to P2 and the quantity consumed to rise to Q2. A drop in the interest rate shifts the supply curve to S3, leading to an increase in price to P3 and a decrease in consumption to Q3.
The supply of an exhaustible resource such as oil is thus governed by its current price, its expected future price, and the interest rate. An increase in the expected future price—or a reduction in the interest rate—reduces the supply of oil today, preserving more for future use. If owners of oil expect lower prices in the future, or if the interest rate rises, they will supply more oil today and conserve less for future use. This relationship is illustrated in Figure 13.8 “Future Generations and Exhaustible Natural Resources”. The current demand D for these services is given by their marginal revenue product (MRP) . Suppose S 1 reflects the current marginal cost of extracting the resource, the prevailing interest rate, and expectations of future demand for the resource. If the interest rate increases, owners will be willing to supply more of the natural resource at each price, thereby shifting the supply curve to the right to S 2 . The current price of the resource will fall. If the interest rate falls, the supply curve for the resource will shift to the left to S 3 as more owners of the resource decide to leave more of the resource in the earth. As a result, the current price rises.
Resource Prices Over Time
Since using nonrenewable resources would seem to mean exhausting a fixed supply, then one would expect the prices of exhaustible natural resources to rise over time as the resources become more and more scarce. Over time, however, the prices of most exhaustible natural resources have fluctuated considerably relative to the prices of all other goods and services. Figure 13.9 “Natural Resource Prices, 1980–2007” shows the prices of four major exhaustible natural resources from 1980 to 2007. Prices have been adjusted for inflation to reflect the prices of these resources relative to other prices.
During the final two decades of the twentieth century, exhaustible natural resource prices were generally falling or stable. With the start of the current century, their prices have been rising. In short, why do prices of natural resources fluctuate as they do? Should the process of continuing to “exhaust” them just drive their prices up over time?
Figure 13.9. Natural Resource Prices, 1980–2007. The chart shows changes in the prices of five exhaustible resources—chromium, copper, nickel, tin, and tungsten (relative to the prices of other goods and services)—from 1890–2003. Sources: U.S. Bureau of the Census, Statistical Abstract of the United States, online; U.S. Energy Information Administration, Annual Energy Review, online.
In setting their expectations, people in the marketplace must anticipate not only future demand but future supply as well. Demand in future periods could fall short of expectations if new technologies produce goods and services using less of a natural resource. That has clearly happened. The quantity of energy—which is generally produced using exhaustible fossil fuels—used to produce a unit of output has fallen by more than half in the last three decades. At the same time, rising income levels around the world, particularly in China and India over the last two decades, have led to increased demand for energy.
Supply increases when previously unknown deposits of natural resources are discovered and when technologies are developed to extract and refine resources more cheaply. Figure 13.10 “An Explanation for Falling Resource Prices” shows that discoveries that reduce the demand below expectations and increase the supply of natural resources can push prices down in a way that people in previous periods might not have anticipated. This scenario explains the fall in some prices of natural resources in the latter part of the twentieth century. To explain the recent rise in exhaustible natural resources prices, we can say that the factors contributing to increased demand for energy and some other exhaustible natural resources were outweighing the factors contributing to increased supply, resulting in higher prices—a scenario opposite to what is shown in Figure 13.10 “An Explanation for Falling Resource Prices”. This upward trend began to reverse itself again in late 2008, as the world economies began to slump.
Figure 13.10. An Explanation for Falling Resource Prices. Demand for resources has increased over time from D1 to D2, but this shift in demand is less than it would have been (D3) if technologies for producing goods and services using less resource per unit of output had not been developed. Supply of resources has increased from S1 to S2 as a result of the discovery of deposits of natural resources and/or development of new technologies for extracting and refining resources. As a result, the prices of many natural resources have fallen.
Will we ever run out of exhaustible natural resources? Past experience suggests that we will not. If no new technologies or discoveries that reduce demand or increase supply occur, then resource prices will rise. As they rise, consumers of these resources will demand lower quantities of these resources. Eventually, the price of a particular resource could rise so high that the quantity demanded would fall to zero. At that point, no more of the resource would be used. There would still be some of the resource in the earth—it simply would not be practical to use more of it. The market simply will not allow us to “run out” of exhaustible natural resources.
Renewable Natural Resources
As is the case with exhaustible natural resources, our consumption of the services of renewable natural resources can affect future generations. Unlike exhaustible resources, however, renewable resources can be consumed in a way that does not diminish their stocks.
Carrying Capacity and Future Generations
The quantity of a renewable natural resource that can be consumed in any period without reducing the stock of the resource available in the next period is its carrying capacity. Suppose, for example, that a school of 10 million fish increases by 1 million fish each year. The carrying capacity of the school is therefore 1 million fish per year—the harvest of 1 million fish each year will leave the size of the population unchanged. Harvests that exceed a resource’s carrying capacity reduce the stock of the resource; harvests that fall short of it increase that stock.
As is the case with exhaustible natural resources, future generations have a stake in current consumption of a renewable resource. Figure 13.11 “Future Generations and Renewable Resources” shows the efficient level of consumption of such a resource. Suppose Q cap is the carrying capacity of a particular resource and S 1 is the supply curve that reflects the current marginal cost of utilizing the resource, including costs for the labor and capital required to make its services available, given the interest rate and expected future demand. The efficient level of consumption in the current period is found at point E, at the intersection of the current period’s demand and supply curves. Notice that in the case shown, current consumption at Q 1 is less than the carrying capacity of the resource. A larger stock of this resource will be available in subsequent periods than is available now.
Figure 13.11. Future Generations and Renewable Resources. The efficient quantity of services to consume is determined by the intersection S1 and the demand curve D. This intersection occurs at point E at a quantity of Q1. This lies below the carrying capacity Qcap. An increase in interest rates, however, shifts the supply curve to S2. The efficient level of current consumption rises to Q2, which now exceeds the carrying capacity of the resource.
Now suppose interest rates increase. As with nonrenewable resources, higher interest rates shift the supply curve to the right, as shown by S 2 . The result is an increase in current consumption to Q 2 . Now consumption exceeds the carrying capacity, and the stock of the resource available to future generations will be reduced. While this solution may be efficient, the resource will not be sustained over time at current levels.
If society is concerned about a reduction in the amount of the resource available in the future, further steps may be required to preserve it. For example, if trees are being cut down faster than they are being replenished in a particular location, such as the Amazon in Brazil, a desire to maintain biological diversity might lead to conservation efforts.
Economic Rent and The Market for Land
We turn finally to the case of land that is used solely for the space it affords for other activities—parks, buildings, golf courses, and so forth. We shall assume that the carrying capacity of such land equals its quantity.
Figure 13.12. The Market for Land. The price of a one-acre parcel of land is determined by the intersection of a vertical supply curve and the demand curve for the parcel. The sum paid for the parcel, shown by the shaded area, is economic rent.
The supply of land is a vertical line. The quantity of land in a particular location is fixed. Suppose, for example, that the price of a one-acre parcel of land is zero. At a price of zero, there is still one acre of land; quantity is unaffected by price. If the price were to rise, there would still be only one acre in the parcel. That means that the price of the parcel exceeds the minimum price—zero—at which the land would be available. The amount by which any price exceeds the minimum price necessary to make a resource available is called economic rent.
The concept of economic rent can be applied to any factor of production that is in fixed supply above a certain price. In this sense, much of the salary received by Brad Pitt constitutes economic rent. At a low enough salary, he might choose to leave the entertainment industry. How low would depend on what he could earn in a best alternative occupation. If he earns $30 million per year now but could earn $100,000 in a best alternative occupation, then $29.9 million of his salary is economic rent. Most of his current earnings are in the form of economic rent, because his salary substantially exceeds the minimum price necessary to keep him supplying his resources to current purposes.
KEY TAKEAWAYS
- Natural resources are either exhaustible or renewable.
- The demand for the services of a natural resource in any period is given by the marginal revenue product of those services.
- Owners of natural resources have an incentive to take into account the current price, the expected future demand for them, and the interest rate when making choices about resource supply.
- The services of a renewable natural resource may be consumed at levels that are below or greater than the carrying capacity of the resource.
- The payment for a resource above the minimum price necessary to make the resource available is economic rent.
Case in Point: World Oil Dilemma
The world is going to need a great deal more oil. Perhaps soon.
The International Energy Agency, regarded as one of the world’s most reliable in assessing the global energy market, says that world oil production must increase from 87 million barrels per day in 2008 to 99 million barrels per day by 2015. Looking farther ahead, the situation gets scarier. Jad Mouawad reported in The New York Times that the number of cars and trucks in the world is expected to double—to 2 billion—in 30 years. The number of passenger jetliners in the world will double in 20 years. The IEA says that the demand for oil will increase by 35% by 2030. Meeting that demand would, according to the Times , require pumping an additional 11 billion barrels of oil each year—an increase of 13%.
Certainly some in Saudi Arabia, which holds a quarter of the world’s oil reserves, were sure it would be capable of meeting the world’s demand for oil, at least in the short term. In the summer of 2005, Peter Maass of The New York Times reported that Saudi Arabia’s oil minister, Ali al-Naimi, gave an upbeat report in Washington, D.C. to a group of world oil officials. With oil prices then around $55 a barrel, he said, “I want to assure you here today that Saudi Arabia’s reserves are plentiful, and we stand ready to increase output as the market dictates.” The minister may well have been speaking in earnest. But, according to the U. S. Energy Information Administration, Saudi Arabia’s oil production was 9.6 million barrels per day in 2005. It fell to 8.7 million barrels per day in 2006 and to 8.7 million barrels per day in 2007. The agency reports that world output also fell in each of those years. World oil prices soared to $147 per barrel in June of 2008. What happened?
Much of the explanation for the reduction in Saudi Arabia’s output in 2006 and 2007 can be found in one field. More than half of the country’s oil production comes from the Ghawar field, the most productive oil field in the world. Ghawar was discovered in 1948 and has provided the bulk of Saudi Arabia’s oil. It has given the kingdom and the world more than 5 million barrels of oil per day for well over 50 years. It is, however, beginning to lose pressure. To continue getting oil from it, the Saudis have begun injecting the field with seawater. That creates new pressure and allows continued, albeit somewhat reduced, production. Falling production at Ghawar has been at the heart of Saudi Arabia’s declining output.
The Saudi’s next big hope is an area known as the Khurais complex. An area about half the size of Connecticut, the Saudis are counting on Khurais to produce 1.2 million barrels per day beginning in 2009. If it does, it will be the world’s fourth largest oil field, behind Ghawar and fields in Mexico and Kuwait. Khurais, however, is no Ghawar. Not only is its expected yield much smaller, but it is going to be far more difficult to exploit. Khurais has no pressure of its own. To extract any oil from it, the Saudis will have to pump a massive amount of seawater from the Persian Gulf, which is 120 miles from Khurais. Injecting the water involves an extraordinary complex of pipes, filters, and more than 100 injection wells for the seawater. The whole project will cost a total of $15 billion. The Saudis told The Wall Street Journal that the development of the Khurais complex is the biggest industrial project underway in the world. The Saudis have used seismic technology to take more than 2.8 million 3-dimensional pictures of the deposit, trying to gain as complete an understanding of what lies beneath the surface as possible. The massive injection of seawater is risky. Done incorrectly, the introduction of the seawater could make the oil unusable.
Khurais illustrates a fundamental problem that the world faces as it contemplates its energy future. The field requires massive investment for an extraordinarily uncertain outcome, one that will only increase Saudi capacity from about 11.3 million barrels per day to 12.5.
Sadad al-Husseini, who until 2004 was the second in command at Aramco and is now a private energy consultant, doubts that Saudi Arabia will be able to achieve even that increase in output. He says that is true of the world in general, that the globe has already reached the maximum production it will ever achieve—the so-called “peak production” theory. What we face, he told The Wall Street Journal in 2008, is a grim future of depleting oil resources and rising prices.
Rising oil prices, of course, lead to greater conservation efforts, and the economic slump that took hold in the latter part of 2008 has led to a sharp reversal in oil prices. But, if “peak production” theory is valid, lower oil prices will not persist after world growth returns to normal. This idea is certainly one to consider as we watch the path of oil prices over the next few years.
Self Check: Factors of Production
Answer the question(s) below to see how well you understand the topics covered in the previous section. This short quiz does not count toward your grade in the class, and you can retake it an unlimited number of times.
You’ll have more success on the Self Check if you’ve completed the ten Readings in this section.
Use this quiz to check your understanding and decide whether to (1) study the previous section further or (2) move on to the next section.
- Principles of Microeconomics Section 13.3. Authored by : Anonymous. Located at : http://2012books.lardbucket.org/books/microeconomics-principles-v1.0/s16-02-interest-rates-and-capital.html . License : CC BY-NC-SA: Attribution-NonCommercial-ShareAlike
Thank you for visiting nature.com. You are using a browser version with limited support for CSS. To obtain the best experience, we recommend you use a more up to date browser (or turn off compatibility mode in Internet Explorer). In the meantime, to ensure continued support, we are displaying the site without styles and JavaScript.
- View all journals
- My Account Login
- Explore content
- About the journal
- Publish with us
- Sign up for alerts
- Open access
- Published: 29 March 2019
Over-exploitation of natural resources is followed by inevitable declines in economic growth and discount rate
- Adam Lampert ORCID: orcid.org/0000-0001-8115-6688 1 , 2
Nature Communications volume 10 , Article number: 1419 ( 2019 ) Cite this article
95k Accesses
72 Citations
15 Altmetric
Metrics details
- Environmental economics
- Sustainability
A major challenge in environmental policymaking is determining whether and how fast our society should adopt sustainable management methods. These decisions may have long-lasting effects on the environment, and therefore, they depend critically on the discount factor, which determines the relative values given to future environmental goods compared to present ones. The discount factor has been a major focus of debate in recent decades, and nevertheless, the potential effect of the environment and its management on the discount factor has been largely ignored. Here we show that to maximize social welfare, policymakers need to consider discount factors that depend on changes in natural resource harvest at the global scale. Particularly, the more our society over-harvests today, the more policymakers should discount the near future, but the less they should discount the far future. This results in a novel discount formula that implies significantly higher values for future environmental goods.
Similar content being viewed by others
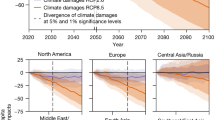
The economic commitment of climate change
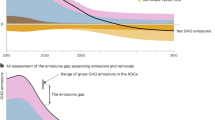
The carbon dioxide removal gap
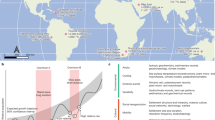
Frequent disturbances enhanced the resilience of past human populations
Introduction.
The exploitation of ecosystems by humans has long-lasting consequences for the future provision of natural resources and ecosystem services 1 , 2 . This may negatively affect the provision of food, increase health hazards and risks of natural disasters, and more. Degraded ecosystems may be slow to recover or may not recover naturally even after their exploitation stops 3 , 4 , 5 . Consequently, the availability of natural resources such as food, clean air, and other ecosystem services, may be adversely impacted for extended periods if the ecosystems providing these resources become degraded. For example, the emission of greenhouse gases may affect the global climate for centuries 6 , 7 ; invasive species and diseases may irreversibly damage ecosystems 8 , 9 ; and the non-sustainable harvest of fisheries and forests may leave these systems degraded for decades 2 , 4 , or even lead to their irreversible and permanent degradation 3 , 10 . Since natural resources are limited, it has been widely recognized that a transition to sustainable harvest is necessary 11 . What the optimal pathway and speed are for this transition, however, constitute the focus of an ongoing debate. For example, it has been suggested that an abrupt transition may slow economic growth in developing countries and may negatively affect production 12 , and that rapid emission cuts may create energy deficits before we manage to develop viable substitutes 13 .
Determining the optimal strategy for the adoption of sustainable management over time requires cost-benefit analyses. A common approach is to consider a social planner whose objective is to maximize social welfare 14 , 15 , 16 . This is often formalized as maximizing a net present value,
where B ( t ) is the benefit minus the cost (in units of consumption) due to both the management and the environment at time t , and Δ( t ) is the cumulative discount. In turn, the discount factor, exp(−Δ( t )), is the number of units of some good or currency needed at present to compensate for the lack of one unit at time t . The rationale behind discounting is that the objective of our society is to maximize welfare rather than net consumption. In turn, if society is going to be wealthier in the future, then one unit of consumed goods in the future may add less to welfare than the same unit today 14 , 15 , 16 .
Accurate discounting is particularly important for environmental policies in which the resultant damages are long-term, such as policies concerning climate change and provision of natural resources 6 , 17 , 18 . Specifically, a small difference in the discount may lead to a large difference in estimates of long-term environmental cost. For example, consider no changes in prices and a constant annual discount rate, δ ≡ dΔ/d t . Then, if the cost due to losing some good today is $1M, then the cost due to losing the exact same good (no depreciation) 100 years from now is ~$50K if δ = 3%, and only ~$2.5K if δ = 6%. Therefore, even the best estimates of environmental damages may lead to an inadequate policy if we are unable to accurately convert future costs to their present-equivalent dollar value.
The central role that discounting plays in the valuation of natural resources has led to extensive debates over the value that policymakers should use for the discount rate and over how this value varies over time. Specifically, the small values given to future environmental goods due to discounting may contradict our intuition that our society should sustain our planet’s ecosystems for future generations. One major debate followed the publication of the Stern report 6 , which used a discount rate that is smaller than those used in previous major assessments, and consequently, argued for radical emission cuts. The bulk of the criticism 19 has focused on which discount rate should policymakers use (not on the comprehensive cost assessments). Also, several authors 16 , 20 , 21 , 22 , 23 proposed that policymakers should use a discount rate that declines over time, and they showed that this is justified if future economic growth is uncertain. Another mechanism that could affect the discount rate is a large perturbation that significantly affects social welfare 24 , 25 , such as an environmental degradation that may occur due to climate change or over-harvesting 26 , 27 , 28 , 29 . Particularly, several authors showed that global changes in the provision of non-substitutable natural resources might affect their relative prices 30 , 31 and the discount rate 25 , 32 . Nevertheless, these authors considered the changes in the provision of natural resources as given, while the long-term consequences of harvesting on economic growth and discount rate remain largely unknown.
In this paper, we examine how the discount rate and factor are affected by large changes in the harvest methods used at the global scale, such as the transition from over-harvesting to harvesting sustainably. Specifically, the decline in the provision of natural resources due to the future transition might be so large that it will significantly affect social welfare and economic growth. In turn, since discount rates depend on welfare and growth, this means that the discount rate itself could be affected. Revealing harvest-induced changes in the discount will provide policymakers with better evaluations of long-term benefits and costs, thereby enabling them to improve long-term environmental policies. We focus on the harvest of renewable resources in a broad sense, where non-sustainable harvest suppresses the future provision of the resource or the ecosystem service. Examples include the over-harvesting of fish and timber that degrades fisheries and forests 10 , and non-sustainable agriculture and land-use that make future land-use less effective 33 , 34 . We show that over-harvesting temporarily keeps the discount rate higher, but is followed by a period of lower discount rates during the same period in which society makes the transition to sustainable harvesting. Specifically, during the transition, the rates of economic growth and discount could be much lower than their rates before and after the transition. Therefore, the more our society over-harvests natural resources today, the more policymakers should discount the near future, but the less they should discount the far future. Furthermore, we prove a theorem implying that postponing or slowing the transition to sustainable harvesting cannot prevent the ultimate declines in the cumulative discount. Accordingly, we develop a discount formula that incorporates the changes in the harvest methods, which, in turn, dictates significantly higher net costs due to long-lasting environmental damages.
Theoretical framework
We consider a social welfare function, U T , that depends on the provision of some natural resource at the global scale, f ( t ), and on the consumption of the other goods, including manufactured goods, c ( t ) (Methods, Eq. 4 ). In turn, the dynamics of the c ( t ) and f ( t ), together with U T , determine the social rate of discount, δ ( t ), which specifies the rate at which goods should be discounted by a social planner whose objective is to maximize social welfare 15 , 16 , 35 . To define the social rate of discount (hereafter, the discount rate), we adopt a well-established framework 12 , 14 , 16 , 32 , 36 , 37 and we assume that it is given by the rate of decline in the marginal contribution of consumption to social welfare (consumption rate of discount). Specifically, we consider a given currency unit, a dollar, that enables the consumption of exactly με units of the natural resource and (1 − μ ) ε units of the other goods, where 0 ≤ μ ≤ 1 and ε is very small. Accordingly, the discount factor at time t is given by the number of dollars needed at present to compensate for a lack of one dollar at time t . Note that the choice of μ does not affect the value given to future goods, and therefore, it does not affect the policy and/or the management decisions; rather, μ determines the units and it affects only the relative role of the discount factor and the prices in determining the value of future goods 36 , 37 . In turn, we show that this implies that the discount rate, δ ( t ), and the cumulative discount \({\mathrm{\Delta }}(t) = {\int}_0^t \delta \left( {t\prime } \right){\mathrm{d}}t\prime\) , are given by Eq. 5 , and the prices of the natural resource and of the other goods are given by Eq. A10 (Methods and Supplementary Note 1). Specifically, the discount rate and the prices depend on the substitutability of the natural resource and the other goods, which is incorporated in the social welfare function. In Supplementary Note 2, we derive specific expressions for the discount rate and for the prices in two cases, one in which the natural resource and the other goods are non-substitutable (Eqs. B5 , B9 ), and one in which they are partially substitutable (Eqs. B12 , B15 , B16 ).
In turn, the novel part of this study comes from endogenizing the dynamics of c ( t ) and f ( t ) by modeling how they depend on the harvest methods used globally (see Methods). This allows us to examine how the discount factor and the prices depend on changes in harvest methods. We assume that, if the harvest methods do not change, then c ( t ) and f ( t ) increase exponentially at fixed rates, g c and g f , respectively, due to exogenous factors such as technological developments and exogenous environmental changes; however, changes in the patterns of harvest may affect c ( t ) and f ( t ), thereby affecting the discount rate over time (see Methods). This approach builds on and generalizes previous studies that considered f ( t ) and c ( t ) that grows exponentially irrespective of the harvest 32 , 37 . Specifically, note that c ( t ) and f ( t ) characterize the total provision of the goods at the global scale, and accordingly, we consider a large ecosystem that comprises a large number of distinct regions (Fig. 1 ). This ecosystem may be, for example, the entire planet’s aquatic ecosystem, where each region is some local fishery providing fish; the forest area on a given continent, where each region is a single forest providing timber; or the area that can be used for agriculture worldwide, where each region is a local geographic area comprised of agricultural fields. We are interested in the long-lasting effects of harvesting on the provision of the natural resource, and therefore, we focus on irreversible degradations of the ecosystem, rather than on temporary fluctuations of the resource stock. These degradations may occur, for example, if some ecosystem services are permanently lost 5 or if the ecosystem that provides the renewable resource collapses or undergoes an irreversible regime shift in some of its regions, such as occurs in eutrophication and deforestation 3 , 4 , 10 . We assume that higher rates of non-sustainable harvest (higher H n ) result in a greater provision of the natural resource at the time of harvest but also result in a higher degradation of the ecosystem (Eq. 6 , see Methods). Specifically, we assume that a given portion of the global ecosystem, H ( t ), is being harvested in year t , while some portion of the ecosystem, H n ( t ), becomes degraded during that year due to non-sustainable harvest, and cannot be used for harvest thereafter (Fig. 1 ). For example, H n ( t ) may characterize the portion of the global fish or timber stock that is lost due to the collapse of fisheries or the irreversible degradation of forests worldwide in year t 38 . For another example, H n ( t ) may characterize the persistent reduction in the yield of crop caused by the degradation of vital ecosystem services and the increase in the persistence of pests 33 , 34 . In turn, H ( t ) and H n ( t ) are determined by the various harvest methods used in the system (see Methods).
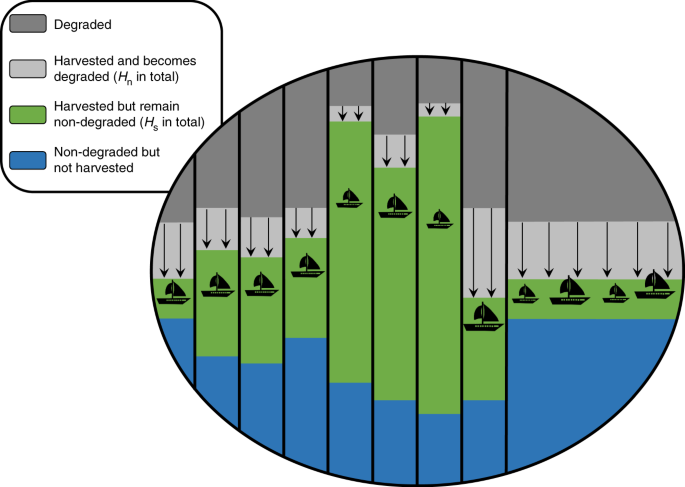
Schematic illustration of the model. Demonstrated is the state of the system at the global scale (e.g., the entire planet’s marine area, forest area, or agricultural area) in a given year. The dark-gray area characterizes the part of the system that is degraded due to former non-sustainable harvesting. The light gray area with the arrows characterizes the part of the system that is being harvested non-sustainably and will be degraded starting next year (total dark-gray area is given by H n ). The green area with the fishing vessels characterizes the part of the system that is being harvested sustainably and will remain non-degraded next year (total green area is given by H s ). (Note that the total area under harvest, H , is given by the green and the light gray areas combined, H = H n + H s ). The blue area characterizes the part of the system that is not degraded but is still not being harvested. We assume that the spatial scale of the system is very large, and therefore, the recovery of the degraded areas due to migrating biota from other regions is negligible and the total degraded area increases over time. Each year, H n and H s are determined by the aggregate management by all the managers. We assume that managers may be subject to different externalities in distinct regions, e.g., some regions are managed by a single manager that dictates the harvest method, while some regions are shared (open-access), and all managers are free to harvest in them (rightmost region). The variables x 1 and x 2 (Eqs. 7 and 8 ) characterize the total non-degraded areas (blue, green, and light gray) in the managed and in the shared regions, respectively
To examine the effect of over-harvesting on the natural resource and on the discount rate, we compare scenarios in which over-harvesting occurs to scenarios in which it does not. We consider two approaches. First, we consider a competitive market approach in which we compare the optimal solution that maximizes social welfare with the solution that emerges in a model of a perfectly competitive market with externalities (Figs. 2 and 3 ). Specifically, the competitive market includes managed regions that have a single manager (e.g., landowner, government), and shared regions in which multiple managers are free to harvest (e.g., open-access) (see Methods). Second, we consider a more general approach in which we compare the dynamics that emerge when the harvest is entirely sustainable with the dynamics that emerge following various ad hoc choices of non-sustainable harvest functions (Theorem and Fig. 4 ).
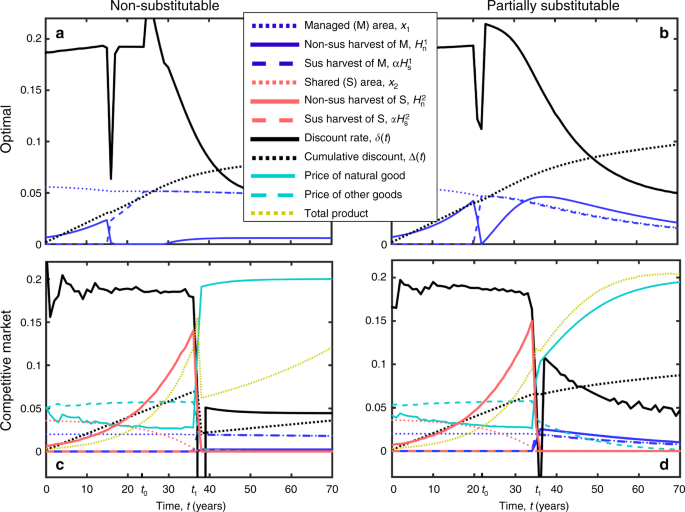
Over-harvesting extends the period during which the discount rate is high, but it is followed by sharp declines in the discount rate and the cumulative discount. Panels a and b demonstrate the optimal harvest of the natural resource from a social planner’s perspective, where the natural resource and the other goods are either non-substitutable ( a , Eq. B2 ) or partially substitutable ( b , Eq. B10 ). In the early stages, harvesting activity increases exponentially and the discount rate is high. Approximately at time t 0 , when harvesting is occurring in the whole system ( H s + H n = x 1 + x 2 ), the total harvest stops increasing and the discount rate decreases. Next, panels c and d demonstrate harvesting in a competitive market in which some of the regions are shared. The parameters and utility functions used in panels c and d are identical to those used in panels a and b , respectively. The period during which the discount rate is high is extended until t = t 1 due to over-harvesting of the natural resource in the shared regions (compare panel a with panel c , and compare panel b with panel d ). However, this period is followed by a rebound in which harvesting declines and the discount rate and the cumulative discount drop. In addition, around t = t 1 , the price of the natural resource increases and the total product decreases. Note that, in accordance with the theorem, the cumulative discount approaches lower values if the harvest is determined by the market. Scaling: the harvest rates are given in (years) −1 , the total non-degraded areas are given in units showing the maximal annual sustainable yield ( ax 1 and ax 2 ), and Δ is given by 100 times the value on the y -axis. The parameter values used are within their realistic ranges (Methods). Parameter values and Source data are provided as a Source Data file
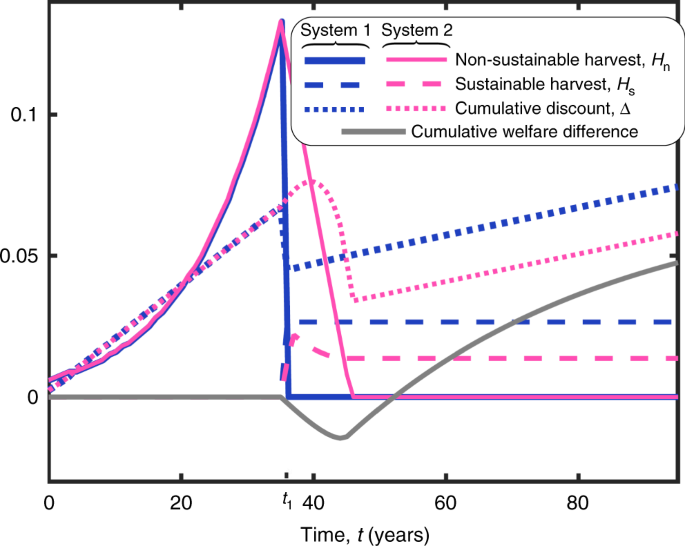
Social welfare and the cumulative discount are ultimately lower if the transition to sustainable harvest is more gradual. Demonstrated are the aggregate non-sustainable harvest, H n ( t ) (solid lines); the aggregate sustainable harvest, H s ( t ) (dashed lines); and the cumulative discount, Δ (dotted lines), for two systems. System 1 (blue) follows the market solution, in which society abruptly stops harvesting non-sustainably at t = t 1 . System 2 (purple) follows the same dynamics until t = t 1 , but then, society gradually shifts to sustainable harvest. The gradual transition postpones the decline in the cumulative discount, but ultimately, it declines to an even lower value than its value in system 1. Moreover, the cumulative welfare, U t , in system 1 is initially smaller, but it ultimately becomes greater compared to system 2 (gray). Harvest rates are given in units of (years) −1 , and Δ is given by 100 times the value on the y -axis. The parameters are the same as in Fig. 2c (Parameter values and Source data are provided as a Source Data file)
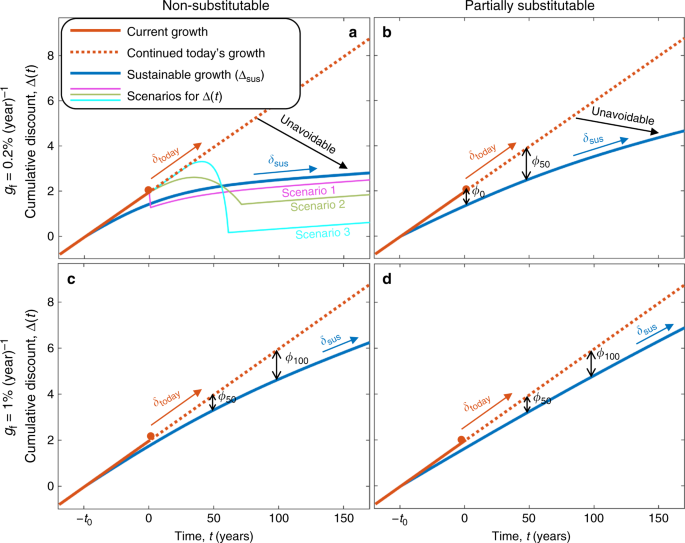
The decline in the cumulative discount is unavoidable (demonstration of the theorem). At some point in time, − t 0 , some planetary boundaries for harvest have been approached, and the rate of discount that would have occurred if managers used only sustainable harvesting has decreased from δ today to δ sus (blue lines, Δ sus ). Nevertheless, due to over-harvesting, the economy grew faster and the cumulative discount, \({\mathrm{\Delta }}(t) = {\int}_0^t \delta (t{\prime}){\mathrm{d}}t{\prime}\) , continued to grow at a higher rate, δ today (solid orange lines), at least until today ( t = 0). In turn, the future value of Δ( t ) depends on the future harvest patterns. If over-harvesting continues, the discount rate might remain close to δ today for several years or decades (dotted orange lines). But in the longer run, according to the theorem, Δ has to decrease below the blue curve that characterizes Δ sus , regardless of how the resource is being harvested. This is also demonstrated for three scenarios in panel a : In scenario 1, the non-sustainable harvest stops today, while in scenarios 2 and 3, the non-sustainable harvest continues for a few decades and then declines gradually. Also, note that Δ sus increases at a rate δ sus , so if one assumes that the discount rate remains δ today for the next τ years and becomes δ sus afterward, then he/she needs to subtract at least ϕ τ to obtain the correct Δ (Eqs. 2 and 3 ). (The value of ϕ τ is demonstrated in Fig. 5 .) We assume that u ( c , f ) is given by Eq. B5 (non-substitutable goods) in panels a and c , and by Eq. B12 (partially substitutable goods) in panels b and d . In turn, the scenarios are calculated for three different choices of H n ( t ), where the dynamics follow Eqs. 6 – 9 with H ( t ) = x 1 ( t ) + x 2 ( t ) for all t . The parameter values used are within their realistic ranges (Methods). Parameter values and Source data are provided as a Source Data file
Over-harvesting is followed by declines in the discount rate
Following the optimal solution in which the harvest functions maximize social welfare, two phases emerge along the time axis (Fig. 2a, b ). In the first phase ( t < t 0 ), c ( t ) is initially small, and the harvest rates are limited due to the direct cost of harvesting (Methods, Eq. 9 ). Over time, as c ( t ) increases, the direct cost plays a less significant role, and the harvest rates increase. Consequently, f ( t ) increases at a rate that is greater than g f , and the discount rate approximately follows Ramsey’s formula. In the second phase ( t > t 0 ), the entire ecosystem is under harvest (either sustainable or non-sustainable). Therefore, the society cannot increase f via harvesting without increasing the non-sustainable harvest (i.e., increasing H n ), which would negatively affect the resource’s future provision. Consequently, the non-sustainable harvest decreases exponentially and c ( t ) and f ( t ) increase at approximately the rates of their technological developments, namely, \(\dot c/c \approx g_{\mathrm{c}}\) and \(\dot f/f \approx g_{\mathrm{f}}\) . This implies that, if g f < g c , the discount rate in the second phase is lower than it was in the first phase (Eqs. B6 , B13 , Supplementary Note 2 ). Note that the optimal solution comprises non-sustainable harvest ( H n > 0) because an increase in f at a given time has a greater effect on welfare than the same increase at a later time; the lower the discount rate, the lower the rate of non-sustainable harvest.
In turn, in the competitive market solution (see Methods), the rate of non-sustainable harvest is higher than the socially optimal rate, namely, the solution exhibits over-harvesting (Fig. 2c, d ). Specifically, the harvest is still primarily sustainable in the managed regions but is non-sustainable in the shared regions. The total area under (non-sustainable) harvest in the shared regions increases over time, and consequently, f ( t ) continues to increase over an extended period of time, which postpones the decline in the discount rate. Eventually, however, at time t = t 1 (Fig. 2 ), the shared regions become entirely degraded and the total rate of non-sustainable harvest declines. In turn, the period during which managers over-harvest ( t < t 1 ) is followed by declines in the discount rate, the cumulative discount (Δ), total production (Eq. A11 ), and the price of manufactured goods (Eq. A10 ). These declines are greater if the magnitude and/or duration of the over-harvesting are greater (e.g., if more regions are shared), and also if the natural and the manufactured goods are non-substitutable. Note that the optimal solution exhibits no declines in economic growth or in Δ because the social planner plans for the forthcoming constraints on the harvest by avoiding over-harvesting in the early stages ( t < t 0 ); in the market solution, managers also take into account the forthcoming decline in f and avoid non-sustainable harvesting in the managed regions prior to time t = t 1 , but they still over-harvest in the shared regions. Also note that, in both the optimal and the market solutions, the harvest functions, as well as c ( t ) and f ( t ), do not depend on μ (only the discount and the prices do).
Decline in the cumulative discount is unavoidable (theorem)
More generally, the following theorem shows that over-harvesting may result in an increase in Δ in the short run, but ultimately, Δ would return to a lower value than it would have had if managers used optimal harvesting or only sustainable harvesting (see proof in Supplementary Note 3 and demonstration in Figs. 3 and 4 ). Specifically, a more gradual transition to using sustainable harvest methods may result in a more gradual decline in Δ, but the ultimate magnitude of the decline must exceed that of the incline in Δ that occurred formerly due to the over-harvesting (Figs. 3 and 4a ). In particular, the theorem shows that the result is robust and does not depend on specific assumptions and parameters. It applies not only in the competitive market model but also in the more general case in which non-sustainable harvest is used instead of more sustainable harvest.
Theorem . Assume that the social welfare, U T , is given by Eq. 4 , where f(t) is given by Eq. 6 , and c(t) is given by Eq. 9 with C 1 = C 2 = constant (Methods). Also, assume that u(c,f) is monotonically increasing and twice differentiable with respect to both of c and f, and all of its second partial derivatives are non-positive (namely, an increase in c or f does not cause another increase to be more beneficial). In addition, we consider g f = 0 and assume that as c \(\rightarrow\) ∞ while f remains fixed, u c /u f \(\rightarrow\) 0 (the price of c approaches 0), u cc /u ff \(\rightarrow\) 0 and u cf /u ff \(\rightarrow\) 0. (Alternatively, we consider g f > 0 and assume that u satisfies the conditions of Lemmas 2B and 3). Finally, we assume that, for sufficiently large t, cu fc /u f and fu ff /u f are monotone with respect to t. (All these assumptions are satisfied if u is given by Eqs. B2 , B10 with η > 1, or various other standard forms 32 , 37 .)
Denote Δ opt as the cumulative discount (Eq. 5 ) that emerges following the optimal harvest. Namely, the non-negative harvest functions maximize social welfare (max U T subject to Eqs. 6 – 9 where T \(\rightarrow\) ∞; see Methods). Next, denote Δ market as the cumulative discount that emerges where the harvest functions are determined if each manager aims to maximize her/his own profit and the non-sustainable harvest may be higher than its socially optimal level (Methods). Then, there exists a time t c such that Δ market ≤ Δ opt for all t ≥ t c . Furthermore, denote Δ sus as the cumulative discount that emerges following optimal harvest while excluding non-sustainable harvest (H n = 0). Then, for any Δ that emerges if non-sustainable harvest occurs (H n (t) > 0) between times t 0 and t 1 , there exists t c > t 1 such that Δ(t c ) ≤ Δ sus (t c ) .
A new discount formula
The theorem shows that an upper bound on Δ( t ) in the long run is given by Δ sus ( t ), the cumulative discount that would have occurred if managers used only sustainable harvest, which increases at a rate given by δ sus (Fig. 4 and Supplementary Note 2). Also, the present value of Δ sus is below Δ because over-harvesting already has occurred prior to today. Specifically, ϕ 0 = Δ(0) − Δ sus (0) reflects the negative shock to Δ that must occur during the transition to sustainable harvest methods due to the prior over-harvesting. It follows that, if t is sufficiently large and δ sus is constant, then
Particularly, if the discount rate has been δ today > δ sus due to non-sustainable harvest during the last t 0 years, and if δ today and δ sus have been constants, then ϕ 0 = ( δ today − δ sus ) t 0 .
The correction to the value of future goods is significant
Next, we calculate the correction to the value of future natural goods as dictated from Eq. 2 . Specifically, we compare the value dictated by the formula to the value dictated by a benchmark policy that assumes that the rate of increase in the provision of the natural resource would remain g c for the next τ years and decrease to g f thereafter 12 . Namely, this benchmark policy ignores the negative shock and simply uses a discount rate given by δ ( t ) = δ today if t ≤ τ and δ ( t ) = δ sus if t > τ . In turn, we would like to calculate the correction to that policy due to the negative shock to Δ. Note that the inevitable decline in the future value of the cumulative discount, ϕ 0 (Eq. 2 ), is what policymakers need to incorporate due to the over-harvesting that has already occurred before t = 0. But if the discount rate remained δ today for the next τ years, until t = τ , then the lower bound on the negative shock, ϕ τ , would be greater than ϕ 0 and given by (Fig. 4 )
This greater shock would compensate for the τ years with the higher discount, such that, in the long run, Δ( t ) would still satisfy Eq. 2 . Note that the shock may be gradual and spread over many years, but this decline in Δ( t ) eventually occurs (Theorem, Figs. 3 and 4 ).
Therefore, this shock implies that the correct discount factor should be greater by a factor of at least exp( ϕ τ ) compared to the one implied by the benchmark policy. Namely, ignoring this shock and simply considering the benchmark policy would result in underestimating the value of future natural goods by a factor of at least exp( ϕ τ ) (Fig. 5 ). In turn, the magnitude of ϕ τ depends on the substitutability of the natural resource and the other goods, as well as on the exogenous growth rates, g c and g f (Supplementary Note 2). For example, if the natural resource is non-substitutable (Eq. B2 ), then δ sus is given by Eq. B5 and ( δ today − δ sus ) \(\rightarrow\) η ( g c − g f ) as t \(\rightarrow\) ∞ (Eq. B7 ). Expressions that result from other utility functions are given in Supplementary Note 2 and in the literature 32 , 37 . These expressions enable us to quantify exp( ϕ τ ) and examine how it depends on the parameters (Fig. 5 ). For example, if g f = 1% year −1 , g c = 2% year −1 36 and τ = 50 years, then the value of future goods before the adjustment is underestimated by a factor greater than two (exp( ϕ τ ) > 2), and this factor is greater if g f is smaller or if τ is larger.
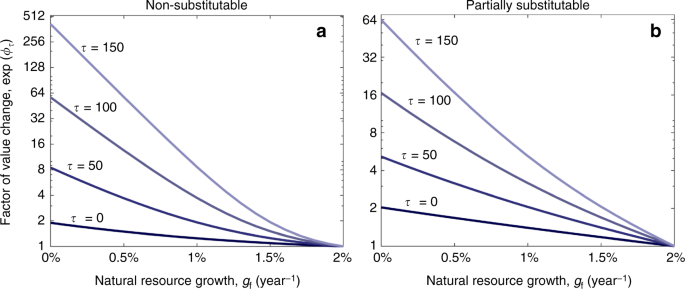
Endogenizing changes in harvest patterns implies a larger discount factor and higher values for future environmental goods. If a policymaker considers a gradual transition to sustainable harvest that would occur within τ years, then he/she may consider a sustainable discount rate, δ sus , starting from year τ . In addition, however, he/she needs to add to Δ another factor, ϕ τ , that accounts for the decline in the cumulative discount that will follow due to over-harvesting prior to time τ (Eqs. 2 , 3 and Fig. 4 ). This factor may impose significantly higher values on future goods, e.g., over two times higher if τ = 50 years and g f = 1% year −1 (exp( ϕ τ ) > 2 in both panels a and b ) and even significantly higher for higher values of τ or lower values of g f . However, if the long-term provision of the natural resource continues to increase at the same rate as the other goods, i.e., g f = g c = 2% year −1 , then δ sus = δ today and ϕ τ = 0 (Eq. 3 ). The other parameter values are the same as in Fig. 4 (Parameter values and Source data are provided as a Source Data file)
After over-harvesting for decades, many societies around the world are beginning to transition to sustainable environmental management practices and sustainable harvest methods 11 . Our study shows that the transition to sustainable harvest methods after a period of over-harvesting is expected to result in a decline in social welfare, economic growth, and the discount rate. In particular, we show that the discount rate, or the social rate of discount, does not decline gradually to its sustainable asymptotic rate; rather, the transition to sustainable harvest may include a period during which the discount rate is far below its asymptotic level (Figs. 2 – 4 and Theorem). Note that several studies suggested that policymakers need to consider discount rates that decline gradually over time due to various mechanisms, including uncertainty in technological growth 16 , 20 , 21 , 22 , 23 , slowdown in technological development due to environmental degradation 27 , 28 , and declining production due to decline in the exploitation of natural resources 12 . In contrast, we showed here that the transition to sustainable harvest imposes a sharper, non-gradual decline in the cumulative discount (Figs. 2 – 4 ). The mechanism underlying this sharper decline is that the rate of increase in the provision of natural resources not only slows down, but must at some point become lower than it would be if over-harvesting had never occurred. In turn, social welfare depends on the provision of natural resources, and therefore, a decline in their provision implies a lower discount rate.
Our results also suggest that the calculations of the discount factor in the long run should not rely on simple extrapolations of the discount rates in the short run. Specifically, over-harvesting might continue for a couple of decades, which may keep the provision of natural resources high in the short run, but will ultimately result in an even lower provision of these resources. Therefore, continued over-harvesting may justify considering higher discount rates in the short run, but it also necessitates discounting the long run less (Fig. 4a ). Ignoring the harvest-induced decline in the discount rate not only falsifies cost-benefit analyses, it also creates a bias: Over-harvesting increases the discount rate in the short run, which might unjustifiably bias the expectations of policymakers to anticipate higher future discount rates, which, in turn, is used to justify further exploitation. (This may also explain why policymakers should consider lower discount rates in the long run although there is no clear evidence that the rate of return on capital will decline during the next 30–40 years 15 .)
To correct for this bias and account for the future decline in the cumulative discount, we developed a new discount formula (Eqs. 2 and 3 ), which provides a simple way to estimate the increase in the present value of future goods due to the transition to sustainable harvest methods. Specifically, policymakers need to consider a cumulative discount, Δ( t ) (Eq. 1 ), that is lower in the long run due to its decline during the transition to sustainable harvest. Although further over-harvesting may postpone the timing of the decline, we prove in the theorem that the decline eventually comes with a rebound as Δ( t ) decreases even further: The more our society over-harvests, the lower Δ( t ) ultimately becomes. Therefore, the expected decline in the cumulative discount must be at least as large as its former increase due to over-harvesting (Eq. 2 , Fig. 4 ). In turn, this former increase is given by Eq. 3 . The correction to discounting suggested by our formula is significant (Fig. 5 ), where adjustments of the order of magnitude implied by the formula suggest significant changes in climate policy, including significant emission cuts 6 , 36 .
Note that the effect of harvest on discounting should be considered in addition to (not instead of) changes dictated by various other mechanisms and considerations. In particular, there is a controversy over the value of the rate of pure time preference, ρ , that should be used in environmental policies; some authors argue that policymakers should determine ρ based on individual’s preferences ( ρ ≈ 3% year −1 ), but others argue that policymakers should use ρ ≈ 0 based on considerations of intergenerational equity 6 , 16 , 19 , 39 . The value of ϕ τ , however, does not depend on the value of ρ and should be subtracted from Δ regardless of that choice. Similarly, uncertainty about technological development may imply that policymakers need to consider δ sus that declines over time 16 , 20 , 21 , 22 , 23 , which implies another decline in the cumulative discount on top of the one suggested here. Also, note that the future values of natural resources do not depend on the proportion given to their consumption in the currency unit, μ . Specifically, their future values do not depend on whether they are accounted for as market or as non-market goods. Nevertheless, μ does affect the relative weights given to the discount factor and to the prices of natural resources in determining the resources’ future values 32 , 36 , 37 . Specifically, ignoring the role of non-market natural resources in economic growth (considering a small μ ) would imply that a change in the provision of these resources has a larger effect on their prices but a smaller effect on the discount factor (Supplementary Note 1). Therefore, focusing on the inevitable increase in the price of natural resources following their over-harvesting would result in the same conclusions and present an alternative approach to the one presented here. In particular, the adjustment exp( ϕ τ ) (Fig. 5 ) is due to the change in the discount factor, while the complementary change in the price (Fig. 2 ) introduces another adjustment to the future value of natural resources 36 . The total adjustment due to changes in both discount and prices does not depend on the choice of μ , and would be ≥exp( ϕ τ ). The significant effect that the global transition to sustainable harvest has on the future value of natural resources suggests that climate policies should be determined jointly with other environmental policies.
Model overview
We begin with describing a well-established framework 32 , 36 , 37 that specifies how social welfare and the discount rate depend on the provision of the natural resource over time, f ( t ), and on the consumption of other goods over time, c ( t ). Next, we specify how harvest at the global scale affects the dynamics of f ( t ) and c ( t ) (which would grow exponentially if the harvest functions are fixed). We complete the model by describing how the harvest strategies are determined by the various managers in a competitive market.
Model of social welfare and the discount rate
We consider a social welfare function that is given by the widely-used form 12 , 32 , 36 , 37
where u ( c , f ) is the instantaneous utility that increases as c and f increase (Table 1 ), ρ is a constant rate of pure time preference, and T is a time horizon (we are interested in the limit T \(\rightarrow\) ∞). The distinction between the provision or consumption of the natural resource, f ( t ), and that of the other goods, c ( t ) is necessary here because, if the natural resource and the other goods are not entirely substitutable and the ratio between them varies over time, then social welfare depends on the ratio between c and f over time and cannot be written as a function of a single variable 29 . In turn, the substitutability is determined by the form of u 12 , 29 , 37 . For example, the goods may be non-substitutable, characterized by separable utility functions (Supplementary Note 2, Eq. B2 ), if one good cannot compensate for the lack of the other good (e.g., many cars cannot compensate for a lack of food). Alternatively, the goods may be partially substitutable (Eq. B10 ) if a sufficient amount of one good may compensate for the lack of the other good (e.g., many carrots can compensate for the lack of fish).
In turn, note that there are several candidates for quantifying the social rate of discount 15 , including the consumption rate of discount and the social and private rates of return to investment. These three quantities are closely-related, and, in a perfectly competitive market, they become equal and reflect the marginal productivity of capital. In this study, as in numerous related studies 12 , 14 , 16 , 32 , 36 , 37 , the focus is on the consumption rate of discount, which is the rate of decline in the marginal contribution of consumption to social welfare. In other words, the corresponding discount factor specifies how many units of consumption added at present would have the same effect on social welfare as a single unit added at time t . In turn when the welfare depends on multiple goods, the discount may depend on the particular good that the policymaker considers 31 , 36 , 37 . (This simply reflects the relative price changes of the goods.) Therefore, to define discount in our system, we consider a small, marginal perturbation to both c and f . Specifically, we consider a given currency unit, a dollar, that allows the consumption of exactly με units of the natural resource and (1 − μ ) ε units of the other goods, where 0 ≤ μ ≤ 1 and ε ≪ c (0), f (0). Accordingly, we define the discount factor at time t as the number of dollars needed at present to compensate for a lack of one dollar at time t . This implies that the discount rate, δ ( t ), is given by (Supplementary Note 1)
where subscripts in this equation denote partial derivatives and the discount factor is given by exp(−Δ). The right side of Eq. 5 , without the term ρ , is due to the change in the marginal contribution of c and f to social welfare. (Note that, if μ = 0 and d c / d t = cg c , then Eq. 5 becomes the Ramsey’s discount formula 14 , 16 , δ ( t ) = ηg c + ρ , where η ≡ cu c / u cc .) In turn, if μ reflects the portion in society’s basket of goods allocated to consumption of the natural resource, then our definition is consistent with the way the marginal productivity of capital is measured, and the total product (e.g., GDP) is proportional to the total value of all the goods (Supplementary Note 1 , Eq. A11 ). Alternatively, if we are interested in discounting some climate damage, then we can chose μ to be proportional to the cost that is due to the damage to the natural resource. Note, however, that the choice of μ only determines the units and does not affect the value given to future goods. Specifically, if the proportion of damages to the natural resource differs from μ , then one should consider the changes in relative prices in addition to discounting 36 , 37 . For example, several authors 37 considered a dual discounting framework in which the natural resource is discounted with μ = 0 and the manufactured goods with μ = 1, where the change in the relative price accounts for the difference; this approach is equivalent to the one presented here.
Model of the dynamics and management of the natural resource
Next, we specify how the harvest methods of the renewable natural resource at the global scale determine the dynamics of c ( t ) and f ( t ) (Fig. 1). Note that the aggregate harvest functions at the global scale are determined by the various harvest methods used at the local scale. In turn, at the local scale, a non-sustainable harvest in a given area during a given year yields β units of the natural resource per unit area, but the ecosystem in that area becomes degraded and ceases to yield resources thereafter. In turn, sustainable harvest in a given area yields less resource ( αβ units, where 0 < α < 1 is a constant), but the area remains fully functional for future use. For example, non-sustainable harvest may include aggressive fishing methods that inflict irreversible damage on fish populations and their habitats, while sustainable harvest implies sustaining fish populations and harvest at the fish growth rate, while also using methods that preserve the habitat and the age and size structures of the fish 38 . In turn, the productivity of the natural resource per unit area, β , may increase due to technological developments but may also decrease due to other environmental changes, such as climate change. Accordingly, we assume that β ( t ) = β 0 exp( g f t ), where β 0 = β (0) and g f is the rate of change in productivity. It follows that the total amount of the natural resource harvested globally at time t is given by
where H n is the area that is non-sustainably harvested in year t (becomes degraded and cannot be harvested thereafter), and H s ( t ) is the area that is being sustainably harvested and remain non-degraded in year t ( H s ( t ) = H ( t ) − H n ( t )).
In turn, we distinguish two types of regions: those that have a single manager, and those that are shared such that all managers are free to harvest. Ultimately, the harvest methods used by all managers determine the total areas that become degraded at time t in the managed and in the shared regions at the global scale, \(H_{\mathrm{n}}^1(t)\) and \(H_{\mathrm{n}}^2(t)\) , respectively \(\left( {H_{\mathrm{n}} = H_{\mathrm{n}}^1 + H_{\mathrm{n}}^2} \right)\) . Accordingly, the total non-degraded areas in all managed regions, x 1 , and in all shared regions, x 2 , decrease due to non-sustainable harvest as follows:
Moreover, the harvest functions are constrained by the non-degraded areas:
for all t , where \(H_{\mathrm{s}} = H_{\mathrm{s}}^1 + H_{\mathrm{s}}^2\) .
In turn, we assume that harvest comes with a direct cost as more labor and resources are directed toward harvesting. We incorporate this direct cost as a reduction in c ( t ), which would otherwise grow exponentially at an exogenous rate g c due to technological developments. Specifically, we assume that c ( t ) is given by
where C 1 and C 2 are the direct costs of harvesting (in units of c ), and λ is the ratio between the direct costs of non-sustainable and sustainable harvest.
Model of the competitive market
It remains to specify how the harvest strategies of the managers at the local scale are determined, and how these strategies determine the harvest functions at the global scale, \(H_{\mathrm{s}}^1(t),\hskip 4ptH_{\mathrm{s}}^2(t),\hskip 4ptH_{\mathrm{n}}^1\) , and \(H_{\mathrm{n}}^2(t)\) . We are interested in comparing two types of solutions: The optimal solution that maximizes the social welfare, and the market solution that emerges in a competitive market. The optimal solution is found via the maximization of the social welfare (Eq. 4 ) subject to the constraints given in Eqs. 6 – 9 . In turn, to define the market solution, we consider a competitive market in which each manager aims to maximize her/his own utility. Specifically, we consider a well-established framework in which the market is perfectly competitive, such that, if property rights are defined everywhere and there are no externalities, the market solution coincides with the optimal solution 12 , 14 , 31 , 40 , 41 , 42 . In turn, the market solution depends on the form of the externalities for the various managers, namely, it depends on how non-sustainable harvest by a given manager affects the ecosystem in regions managed by other managers.
To define the externalities, we distinguish between managed regions and shared regions (Fig. 1 ). Each managed region is managed by a single manager who determines the harvest method, which may vary anywhere between using only sustainable methods and using only non-sustainable methods. In turn, the harvest method in a given region determines the portion of the region that is harvested and the rate at which the region becomes degraded (Fig. 1 ). We assume that the management in a given managed region has no externalities as it affects only the degradation level in that region. In turn, the shared regions are managed by a very large number of managers, each of whom is free to harvest without restrictions there. Specifically, we assume that each manager ignores the effect of her/his actions on the future provision of the resource in the shared regions and considers only her/his instantaneous benefit and cost from the harvest. Consequently, the managers have the incentive to increase non-sustainable harvest in the shared regions until the price of the natural resource equals the direct cost of the harvest. These considerations enable us to find the market solution that is given by the unique Nash equilibrium (see the section Numerical methods). In particular, the perfectly competitive market assumption implies that the management in the managed regions is socially optimal under the constraint given by the management in the shared regions. Note that, without shared regions ( x 2 = 0), there are no externalities and the market solution coincides with the optimal solution.
Numerical methods
The numerical results showing the optimal and market solutions are demonstrated in Figs. 2 and 3 , system 1. The optimal solution is given by the unique set of non-negative aggregate harvest functions, \(H_{\mathrm{s}}^1(t),\hskip 4ptH_{\mathrm{s}}^2(t),\hskip 4ptH_{\mathrm{n}}^1\) , and \(H_{\mathrm{n}}^2(t)\) , that maximize social welfare: max U T (Eq. 4 ) in the limit T \(\rightarrow\) ∞, where c ( t ) and f ( t ) are given by Eqs. 6 and 9 , subject to the constraint given in Eqs. 7 and 8 . (Note that using the social welfare function given in Eq. 4 with a constant ρ , and considering deterministic dynamics of c and f , guarantee that the optimization problem is time consistent and has a unique solution 12 , 37 .) In turn, the market solution is determined by a perfectly competitive market where each manager maximizes her/his own profit. Specifically, consider the set of non-negative harvest functions that maximize utility, max U T (Eq. 4 ) as T \(\rightarrow\) ∞, subject to the constraint given by Eqs. 7a and 8 and the constraint d x 2 /d t = X ( t ). Then, the market harvest is given by the unique solution that satisfies \(X(t) = H_{\mathrm{n}}^2(t)\) (consisteny criterion).
We used algorithms that find the exact solutions provided that the resolutions are sufficiently fine. Specifically, to find the optimal solution numerically, our algorithm uses Stochastic Programming with backward induction (Supplementary Note 4) 43 , 44 . (Note that the model’s dynamics are deterministic but the general method is still called stochastic.) To find the market solution, our algorithm also uses Stochastic Programming to solve for a given value of X . But it finds a solution multiple times, each time for a different value of X , until it finds the solution that satisfies the consisteny criterion. These algorithms are coded in C/C++ and are described in detail in Supplementary Note 4.
In turn, in the results shown in Fig. 3 , system 2, as well as in Figs. 4 and 5 and in the graphical tool, we assume that the dynamics of c and f follow Eqs. 6 – 9 , but we consider harvest functions that are not given by either the optimal solution or the market solution. In Fig. 3 , system 2, we consider harvest functions that follow the market solution until t = t 1 and after t = t 1 + 10, but between these times, the non-sustainable harvest decreases gradually from its maximal level to zero. In Fig. 4 , we calculate Δ sus , which is the cumulative discount that emerges if the harvest is entirely sustainable, namely, H n = 0 and H s = x 1 + x 2 if t > 0. Also, in Fig. 4a , we consider three scenarios in which the non-sustainable harvest is higher in the beginning but eventually approaches zero, while H n + H s = x 1 + x 2 .
After we determine the harvest functions, the functions c ( t ) and f ( t ) are calculated according to Eqs. 6 and 9 . In turn, we calculate the discount rate and the cumulative discount according to Eq. 5 (where the cumulative discount is the integral over time of the discount rate). Specifically, for the case in which only sustainable harvest is used (Δ sus in Fig. 4 ), the discount rates are calculated in Supplementary Note 2 and are given by Eqs. B5 and B12 . The prices are given by Eq. A10 , and the total product is given by Eq. A11 . All of these equations are derived in Supplementary Notes 1 , 2 .
Choice of parameters
The parameter values used for all of the numerical simulations, which are given in the Source Data file, are within their realistic ranges. The rate of technological growth is around 1.5–2.0% year −1 in developed countries and is higher in some developing countries 16 , 45 . In turn, the rate of growth in the yield per unit of sustainable harvest, g f , depends on the specific natural resource, where values that were considered in the literature vary from g c down to much lower (even negative) values 32 , 37 . Next, the value of 0 ≤ a ≤ 1 (unitless) also depends on the particular system. In a fishery, for example, if non-sustainable harvest would imply catching all the fish and sustainable harvest would imply keeping the fish population size fixed, then a would be the growth rate of the fish (i.e., 2% year −1 for large fish and higher rates for smaller fish) 38 ; In agriculture, sustainable management implies the use of environmentally friendly pest control methods and effective water management, which may result in a comparable crop yield ( α ≲ 1), but may be more expensive ( λ > 1) 33 , 34 . In turn, the ratio between c ( t ) and the direct costs, C 1 and C 2 (Eq. 9 ), determines the relative portion of c that is needed per unit of harvest. Specifically, c (and thus the ratio) is initially small but increases due to technological changes. Also, C 1 and C 2 may vary with x 1 and x 2 if the cost varies among regions (e.g., if near-shore regions are depleted, the average direct cost of harvest may increase). Next, note that 0 ≤ μ ≤ 1 (unitless) can be chosen arbitrarily by the policymaker, as it does not affect the harvest strategy and the future value of the natural resource; rather, it determines the currency unit, which, in turn, determines the relative role of the discount and the price in determining the future value of the natural resource. A reasonable choice would be the portion in the basket of goods of the natural resource (e.g., the portion of agricultural products in consumption is ~5% in the United States and is higher in various developing countries), but μ may be higher if non-market goods are incorporated. Finally, a variety of utility functions that incorporate both c and f were suggested in the literature 12 , 32 , 37 , including the two that are used here (Eqs. B2 , B10 ) 12 , where estimates of η vary between 1 and 3 (unitless) 16 , 41 , 45 , and suggested values for ρ varies between 0 and 3% (year −1 ) 6 , 16 , 19 , 45 .
Analytical and theoretical analysis
The general discount formula (Eq. 5 ) is derived in Supplementary Note 1. The discount formulas for the special cases presented in the figures are derived in Supplementary Note 2. The proof of the theorem is given in Supplementary Note 3.
Data availability
No datasets were generated or analyzed during the current study. All the data needed to reproduce the results is given in the paper. In particular, the parameter values used for each figure are given in the Source Data file. These parameter values are taken from the references that are cited in the Methods section.
Code availability
The algorithm that we used for finding the optimal and market solutions (Figs. 2 and 3 ) is described in Supplementary Note 4. The C/C++ code used for generating Figs. 2 and 3 as well as the Matlab code used for generating Figs. 4 and 5 are available as a supplementary code.
Assessment, M. E. Ecosystems and Human Well-being (Island Press, Washington, DC, 2005).
Groom, M. J., Meffe, G. K. & Carroll, C. R. Principles of Conservation Biology (Sinauer Associates, Sunderland, 2006).
Scheffer, M. & Carpenter, S. R. Catastrophic regime shifts in ecosystems: linking theory to observation. Trends Ecol. & Evol. 18 , 648–656 (2003).
Article Google Scholar
Folke, C. et al. Regime shifts, resilience, and biodiversity in ecosystem management. Annu. Rev. Ecol., Evol., Syst. 35 , 557–581 (2004).
Moreno-Mateos, D. et al. Anthropogenic ecosystem disturbance and the recovery debt. Nat. Commun. 8 , 14163 (2017).
Article ADS CAS Google Scholar
Stern, N. H. The Economics of Climate Change: the Stern Review . (Cambridge University Press, Cambridge, 2007).
Solomon, S., Plattner, G.-K., Knutti, R. & Friedlingstein, P. Irreversible climate change due to carbon dioxide emissions. Proc. Natl Acad. Sci. USA 106 , 1704–1709 (2009).
Coomes, D. A., Allen, R. B., Forsyth, D. M. & Lee, W. G. Factors preventing the recovery of New Zealand forests following control of invasive deer. Conserv. Biol. 17 , 450–459 (2003).
Hulme, P. E. Beyond control: wider implications for the management of biological invasions. J. Appl. Ecol. 43 , 835–847 (2006).
Barnosky, A. D. et al. Approaching a state shift in Earth’s biosphere. Nature 486 , 52–58 (2012).
Bringezu, S. & Bleischwitz, R. Sustainable Resource Management: Global Trends, Visions and Policies (Routledge, New York, 2009).
Xepapadeas, A. in Handbook of Environmental Economics (ed. Vincent, J. R. and Mäler, K. G.). (Elsevier, North Holland, 2005).
Chu, S. & Majumdar, A. Opportunities and challenges for a sustainable energy future. Nature 488 , 294–303 (2012).
Ramsey, F. P. A mathematical theory of saving. Econ. J. 38 , 543–559 (1928).
Groom, B., Hepburn, C., Koundouri, P. & Pearce, D. Declining discount rates: the long and the short of it. Environ. Resour. Econ. 32 , 445–493 (2005).
Arrow, K. J. et al. Should governments use a declining discount rate in project analysis? Rev. Environ. Econ. Policy 8 , 145–163 (2014).
Nordhaus, W. D. To slow or not to slow: the economics of the greenhouse effect. Econ. J. 101 , 920–937 (1991).
Arrow, K. J. et al. Determining benefits and costs for future generations. Science 341 , 349–350 (2013).
Nordhaus, W. D. A review of the Stern review on the economics of climate change. J. Econ. Lit. 45 , 686–702 (2007).
Weitzman, M. L. Why the far-distant future should be discounted at its lowest possible rate. J. Environ. Econ. Manag. 36 , 201–208 (1998).
Weitzman, M. L. Gamma discounting. Am. Econ. Rev. 90 , 260–271 (2001).
Weitzman, M. L. A review of the Stern Review on the economics of climate change. J. Econ. Lit. 45 , 703–724 (2007).
Gollier, C. Pricing the Planet’s Future: the Economics of Discounting in an Uncertain World (Princeton University Press, Princeton, 2013).
Van der Zwaan, B. C., Gerlagh, R. & Schrattenholzer, L. Endogenous technological change in climate change modelling. Energy Econ. 24 , 1–19 (2002).
Dietz, S. & Hepburn, C. Benefit–cost analysis of non-marginal climate and energy projects. Energy Econ. 40 , 61–71 (2013).
Dell, M., Jones, B. F. & Olken, B. A. Temperature shocks and economic growth: evidence from the last half century. Am. Econ. J.: Macroecon. 4 , 66–95 (2012).
Google Scholar
Moore, F. C. & Diaz, D. B. Temperature impacts on economic growth warrant stringent mitigation policy. Nat. Clim. Change 5 , 127–131 (2015).
Article ADS Google Scholar
Dietz, S. & Stern, N. Endogenous growth, convexity of damage and climate risk: how Nordhaus’ framework supports deep cuts in carbon emissions. Econ. J. 125 , 574–620 (2015).
Drupp, M. A. Limits to substitution between ecosystem services and manufactured goods and implications for social discounting. Environ. Resour. Econ. 69 , 135–158 (2018).
Neumayer, E. Global warming: discounting is not the issue, but substitutability is. Energy Policy 27 , 33–43 (1999).
Neumayer, E. Weak Versus Strong Sustainability: Exploring the Limits of Two Opposing Paradigms (Edward Elgar Publishing, Cheltenham, 2003).
Hoel, M. & Sterner, T. Discounting and relative prices. Clim. Change 84 , 265–280 (2007).
Brewer, M. J. & Goodell, P. B. Approaches and incentives to implement integrated pest management that addresses regional and environmental issues. Annu. Rev. Entomol. 57 , 41–59 (2012).
Article CAS Google Scholar
Lefebvre, M., Langrell, S. R. & Gomez-y-Paloma, S. Incentives and policies for integrated pest management in Europe: a review. Agron. Sustain. Dev. 35 , 27–45 (2015).
Lind, R. C. in Discounting for Time and Risk in Energy Policy (eds Lind, R. C. et al.) (Resources for the Future, Washington, DC, 1982).
Sterner, T. & Persson, U. M. An even sterner review: Introducing relative prices into the discounting debate. Rev. Environ. Econ. Policy 2 , 61–76 (2008).
Traeger, C. P. Sustainability, limited substitutability, and non-constant social discount rates. J. Environ. Econ. Manag. 62 , 215–228 (2011).
Clark, C. W. Mathematical Bioeconomics: the Mathematics of Conservation (John Wiley & Sons, New Jersey, 2010).
Stern, N. Ethics, equity and the economics of climate change paper 2. Econ. Philos. 30 , 445–501 (2014).
Becker, R. A. & Boyd III, J. H. Capital Theory, Equilibrium Analysis, and Recursive Utility (Wiley-Blackwell, New Jersey, 1997).
Evans, D. J. The elasticity of marginal utility of consumption: estimates for 20 OECD countries. Fisc. Stud. 26 , 197–224 (2005).
Romer D. Advanced Macroeconomics , 4th edn (Mcgraw-Hill, New York, 2011).
Conrad, J. M. & Clark, C. W. Natural Resource Economics: Notes and Problems (Cambridge University Press, Cambridge, 1987).
Clark, C. W. & Mangel, M. Dynamic State Variable Models in Ecology: Methods and Applications (Oxford University Press, Oxford, 2000).
Nordhaus, W. D. Managing the Global Commons: the Economics of Climate Change . (MIT press, Cambridge, MA, 1994).
Download references
Acknowledgements
The author sincerely thanks Charles Perrings for his valuable comments on the paper. The author thanks SAL MCMSC, CLAS and SHESC, ASU, for funding (no. DN5-1057).
Author information
Authors and affiliations.
School of Human Evolution and Social Change, Arizona State University, Tempe, AZ, 85287, USA
Adam Lampert
Simon A. Levin Mathematical, Computational and Modeling Science Center, Arizona State University, Tempe, AZ, 85287, USA
You can also search for this author in PubMed Google Scholar
Contributions
A.L. designed the research, composed the model, conducted the research, and wrote the paper.
Corresponding author
Correspondence to Adam Lampert .
Ethics declarations
Competing interests.
The author declares no competing interests.
Additional information
Journal peer review information : Nature Communications thanks the anonymous reviewers for their contribution to the peer review of this work. Peer reviewer reports are available.
Publisher’s note: Springer Nature remains neutral with regard to jurisdictional claims in published maps and institutional affiliations.
Supplementary information
Supplementary information, peer review file, source data, source data, rights and permissions.
Open Access This article is licensed under a Creative Commons Attribution 4.0 International License, which permits use, sharing, adaptation, distribution and reproduction in any medium or format, as long as you give appropriate credit to the original author(s) and the source, provide a link to the Creative Commons license, and indicate if changes were made. The images or other third party material in this article are included in the article’s Creative Commons license, unless indicated otherwise in a credit line to the material. If material is not included in the article’s Creative Commons license and your intended use is not permitted by statutory regulation or exceeds the permitted use, you will need to obtain permission directly from the copyright holder. To view a copy of this license, visit http://creativecommons.org/licenses/by/4.0/ .
Reprints and permissions
About this article
Cite this article.
Lampert, A. Over-exploitation of natural resources is followed by inevitable declines in economic growth and discount rate. Nat Commun 10 , 1419 (2019). https://doi.org/10.1038/s41467-019-09246-2
Download citation
Received : 08 August 2018
Accepted : 25 February 2019
Published : 29 March 2019
DOI : https://doi.org/10.1038/s41467-019-09246-2
Share this article
Anyone you share the following link with will be able to read this content:
Sorry, a shareable link is not currently available for this article.
Provided by the Springer Nature SharedIt content-sharing initiative
This article is cited by
A comprehensive analysis of the inter-relationships of impact between automotive industry, economic growth, natural resources and environmental degradation: morocco as an example.
- Mohammed El-Khodary
- Amine El Kadri
- Sara Dassouli
Environment, Development and Sustainability (2024)
Impact of LULC changes on hydrological flow regimes and runoff coefficient in Upper Jhelum Basin, India
- Shahid Ul Islam
- Sumedha Chakma
Sustainable Water Resources Management (2024)
Urban water-energy-food nexus in the kitchen and social practices of diet and cooking: implications for household sustainability
- Abubakari Ahmed
Industrial output, services and carbon emissions: the role of information and communication technologies and economic freedom in Africa
- Chinazaekpere Nwani
- Festus Victor Bekun
- Ekpeno L. Effiong
Environment, Development and Sustainability (2023)
Towards sustainable food production and climate change mitigation: an attributional life cycle assessment comparing industrial and basalt rock dust fertilisers
- Eunice Oppon
- S.C. Lenny Koh
- Frank Donkor
The International Journal of Life Cycle Assessment (2023)
By submitting a comment you agree to abide by our Terms and Community Guidelines . If you find something abusive or that does not comply with our terms or guidelines please flag it as inappropriate.
Quick links
- Explore articles by subject
- Guide to authors
- Editorial policies
Sign up for the Nature Briefing newsletter — what matters in science, free to your inbox daily.

Distribution of Natural Resources
Different regions have access to different renewable or nonrenewable natural resources such as freshwater, fossil fuels, fertile soil, or timber based on their geographic location and past geologic processes. Access, or the lack thereof, contributes to a place’s economic development, political relationships, and culture. For example, the Great Plains region of the United States is known for its abundance of fertile soil. As a result, its main industry is agriculture. Corn, soybeans, and wheat are globally exported from this region and serve as the main economy. On the other side of the spectrum, the desert southwestern region of the United States depends on the Central Arizona Project canals to transport water from the Colorado River in order to support agriculture and urban areas. Arizona’s right to use water from this river stems from the Colorado Compact, an agreement established in 1922.
Earth Science, Geology, Geography, Physical Geography, Chemistry
If you're seeing this message, it means we're having trouble loading external resources on our website.
If you're behind a web filter, please make sure that the domains *.kastatic.org and *.kasandbox.org are unblocked.
To log in and use all the features of Khan Academy, please enable JavaScript in your browser.
Middle school Earth and space science - NGSS
Course: middle school earth and space science - ngss > unit 5, natural resources.
- Understand: natural resources
- A natural resource is anything found in nature that can be used by humans.
- There are two types of natural resources: renewable and nonrenewable. Renewable resources can be replaced over human lifetimes. Nonrenewable resources cannot.
- Renewable resources are unevenly distributed around Earth. For example, some areas on Earth are windier than others. As a result, these areas are able to generate wind energy more easily.
- Nonrenewable resources are also unevenly distributed. This is because these resources are formed by geologic processes, which occur in specific places on Earth.
- Human activity can change the distribution of certain resources. For example, building a dam can increase the amount of fresh water in one region and decrease it in another.
Want to join the conversation?
- Upvote Button navigates to signup page
- Downvote Button navigates to signup page
- Flag Button navigates to signup page

- Search Search Please fill out this field.
- US & World Economies
U.S. Natural Resources
6 Natural Resources Found in the U.S.
:max_bytes(150000):strip_icc():format(webp)/HeadshotThomasBrock03.08.20-ThomasBrock-c2ad9cdda2394a0f87cf0a78981172b1.jpeg)
Large Landmass
Coastlines with shipping access.
- Land for Farming
- Water for Agriculture
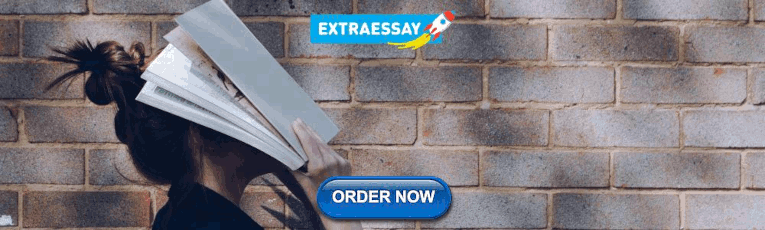
Oil, Coal, and Gas
A unique labor force.
- How Natural Resources Support the Economy
Frequently Asked Questions (FAQs)
The Balance / Hilary Allison
Natural resources are materials from the earth that people use to meet their needs. The United States has an unusual abundance of six natural resources: a large landmass, two expansive coastlines, a wide swath of fertile land, abundant fresh water, huge reserves of oil and coal, and a diverse population.
Learn more about these natural resources and how they boost the U.S. economy.
Key Takeaways
- Six natural resources give the U.S. economy with a superior advantage.
- These include a large landmass, expansive coastlines, fertile land, fresh water, oil and coal, and a diverse population.
- Natural resources make up one of the four factors of production necessary for an economy.
- Without natural resources, there can be no economy.
The geography and geology of the United States provided a tremendous comparative advantage in building its economy. Only Australia and Canada have similar-sized landmasses that aren't bordered by enemies. China's and Russia's landmasses are the same size or larger, but are bordered by enemies, making them subject to invasion. The European Union has a similar size, but not one national government.
America's large landmass under one nation allows economies of scale in government and businesses. This lowers the cost of providing services and products.
America has 95,471 miles of shoreline, including the Great Lakes and outlying U.S. territories and possessions.
The coast contributed $346 billion to the gross domestic product in 2018. It created 3.4 million jobs. These jobs are related to tourism and ocean recreation as well as mineral extraction, boat building, living resources, and marine transportation and construction. The coast created $140 billion in wages for workers.
Countries that are landlocked or have little access to the sea find that both exports and imports are more expensive.
America is fortunate to have a large coastline. Commerce in landlocked countries is dependent upon the whims of another government.
America's large coastline means no hostile governments border it. This allowed the United States to develop peacefully without the need to incur large war costs.
Land for Farming and Agriculture
Unlike Australia and Canada, the United States had temperate climates combined with fertile soil. The early settlers found rich soil on the Great Plains. This is a more than 290,000-square-mile area between the Mississippi River and the Rocky Mountains.
The Plains was a huge basin sculpted out by glaciers during the Great Ice Age. As a result, mountain streams from the Rockies deposited layers of sediment. These streams then cut through the sediment to create plateaus. These large flat areas were untouched by erosion. That created thick sod and productive agriculture.
But the Great Plains is semi-arid, experiencing periodic droughts. The Plains became the breadbasket of the world only after irrigation was put into place. The water came from streams fed by the Rockies.
Water for Agriculture, Life, and More
Lakes, rivers, and streams provide 87% of the water used in America. The electric power industry uses an astonishing 133 billion gallons per day. Water cools electricity-generating equipment, but it is returned. Agricultural irrigation uses 118 billion gallons per day, but it is not returned. Families, businesses, and industries use the rest.
The United States Geological Survey estimates that four states accounted for more than 25% of all water withdrawals in the U.S.: California, Texas, Idaho, and Florida.
America has the world's largest reserves of coal. This abundant source of energy helped fuel U.S. growth during the Industrial Revolution. It fueled steamships and steam-powered railroads. After the Civil War, coke, a derivative of coal, was used to fuel the iron blast furnaces that made steel. Soon after that, coal ran the electricity-generating plants. It still does for many, although that use is declining.
Unlike Canada's shale oil , the United States had huge reserves of oil that were easily accessible. As World War I brewed, the United States converted its coal-burning Navy ships to oil. That made ships faster, extended their range, and allowed easier refueling. Oil was also easily available on the West Coast, allowing the Navy to extend its reach across the Pacific.
Oil made possible many innovations, including cars, trucks, tanks, submarines, and airplanes. Scientists made trinitrotoluene, known as TNT, out of toluene, which they extracted from oil. The United States supplied more than 80% of Allied requirements during World War I.
After the war, oil supplied the power for the internal combustion engine. It also powered the machinery and petrochemicals needed to boost agricultural production.
In 1920, America supplied two-thirds of the world's oil production.
The number of cars registered increased from 3.4 million in 1916 to 23.1 million in 1929. That allowed America to move away from public transit. By 1925, oil accounted for almost one-fifth of U.S. energy consumption. That grew to one-third by World War II.
Other countries only used oil as secondary fuel. It accounted for less than 10% of their energy consumption. When the giant East Texas oil field was discovered in 1930, overproduction became the main issue facing the oil industry.
By 1950, those reserves weren't as cheap. Saudi Arabia and other producers in the Middle East supplied oil more cheaply than U.S. fields could. By 2005, 60% of the oil used in the United States was imported.
In 2011, oil prices were high enough to fund low-cost exploration of U.S. shale oil. By 2020, the U.S. became a net petroleum exporter for the first time since 1949.
America has 44.9 million immigrants , comprising 13.7% of its population by 2019. Most of the people who came to the U.S. throughout its history have had the courage and flexibility needed to survive in a new country. They helped create an innovative culture.
Courage and flexibility spurred early immigrants to continue taking risks once they became citizens.
This cultural diversity is a strength in groups if people remember their common goals. When managed well, diversity brings fresh perspectives based on different experiences. But it takes the willingness to be open-minded and non-judgmental about the value brought by those differences.
President John F. Kennedy was the grandson of Irish immigrants. Kennedy summed it up well when he called America "a society of immigrants, each of whom had begun life anew, on an equal footing. This is the secret of America: a nation of people with the fresh memory of old traditions who dare to explore new frontiers...."
How Resources Support the Economy
Natural resources are one of the four factors of production that are necessary for an economy to begin producing goods. The other three are capital, entrepreneurship, and labor. Capital is the machinery, equipment, and chemicals used in production. Entrepreneurship is the drive to develop an idea into a business. Labor is the workforce.
In a market economy, these components provide the supply that meets the demand from consumers.
If one of the categories is not present, the economy does not exist because production cannot be achieved.
Renewable and Nonrenewable Resources
Natural resources are categorized into two types. The first, renewable resources , are those that are used at a slower rate than they are replaced. These include water, wind, and the sun. Two categories, plants and animals, are considered renewable even though we may be entering the sixth mass extinction.
The second, nonrenewable resources, are those that humanity uses faster than nature can replenish them. These include crude oil, coal, and natural gas, as well as minerals. The sun could be considered a nonrenewable resource because, one day, it will burn out. But most people put it in the renewable category since that won't be for another 5 billion years.
The United States has an affluence of both renewable and nonrenewable natural resources. Most important is its diverse population of different cultures which bring fresh ideas and innovation to business endeavors. These advantages have enabled America to become a major global economic power.
How are U.S. natural resources a source of power?
U.S. natural resources such as coal, oil, and even wind and sun provide literal power in the form of energy generation. But ownership of natural resources provides another sort of power: political, social, and economic power . In the U.S., natural resources can be owned by the government and private individuals. This ownership confers power in the form of wealth and influence.
How much U.S. natural resources are left?
In the U.S., some resources are able to be renewed, such as crops and livestock, as well as solar and wind power. But the nonrenewable resources are finite. Experts from Stanford University have estimated the remaining fossil fuels could be used up in decades: 30 years for oil , 40 years for gas, and 70 years for coal.
National Ocean Service. " How Long Is the U.S. Shoreline? "
Office for Coastal Management, National Oceanic and Atmospheric Administration. " NOAA Report on the U.S. Ocean and Great Lakes Economy ," Page 1.
National Park Service. " Great Plains Province ."
U.S. Geological Survey. " Total Water Use ."
Energy.gov. " Fossil Energy Study Guide: Coal ," Page 3.
David S. Painter. " Oil and the American Century ," Journal of American History .
Congressional Research Service. " U.S. Oil Imports and Exports ," Page 2.
U.S. Energy Information Administration. " Oil and Petroleum Products Explained ."
Migration Policy Institute. " Frequently Requested Statistics on Immigrants and Immigration in the United States ."
U.S. Diplomatic Mission to Germany. “ U.S. Society, A Nation of Immigrants ,”
PhysOrg. " The Sun Won't Die for 5 Billion Years, So Why Do Humans Have Only 1 Billion Years Left on Earth? "
National Resources Revenue Data. " How Revenue Works ."
Millennium Alliance for Humanity and the Biosphere (MAHB). " When Fossil Fuels Run Out, What Then? "
Environment & Natural Resources
How Americans interact with the environment and natural resources impacts everything from the economy to health.
Table of Contents
What is the state of the environment and natural resources in the us.
How is the trend of reducing emissions in the US?
Greenhouse gas emissions
Greenhouse gas emissions by state, energy-related emissions worldwide.
What are the current climate change trends in the US?
Annual average temperature difference by state
How much US land is protected?
Protected lands by state
How many threatened and endangered species are in the US?
Threatened and endangered species
How much water do Americans use?
More on the environment from USAFacts
The government keeps track of information about the environment and how people impact it. This includes data about the weather, which helps us understand climate patterns , and the gases that people release into the air, called greenhouse gases. It also includes information about protecting land and species that are in danger. This page shares some of this information about the environment and natural resources to help answer basic questions about the world around us.
In 2020 , a net total of 5.2 billion metric tons of carbon dioxide (or equivalent) was emitted in the US.
That's 15.8 metric tons per person., emissions in the us peaked in 2007..
Greenhouse gases include a group of gases — primarily carbon dioxide — that are present in the earth's atmosphere that traps heat. Greenhouse gas emissions refer to the amount of gas added by human activity. Fossil fuel burning is the primary cause of emissions, but other activities such as deforestation and industrial processes also create greenhouse gases. After rejoining the Paris Agreement — an international treaty addressing climate change — the US pledged to reduce net greenhouse gas emissions in the US by 50 to 52 percent below 2005 levels by 2030 .
The EPA compiles and calculates emissions data from several sources, including other agencies. It also runs the Greenhouse Gas Reporting Program , requiring large emission sources, fuel and industrial gas supplies, and carbon dioxide injection sites to provide the agency with data.
Since 1990, emissions in the United States have decreased. Every year since 2017, transportation has been the leading emissions sector. This includes 2020 when emissions dropped 10.6% over the previous year due to the pandemic.
In 2020 , Texas had the most greenhouse gas emissions of any state, with a net total of 786.6 million metric tons . Wyoming had the highest greenhouse gas emissions per capita at 145.5 metric tons.
Population size, population density, types of economic activity, and energy sources are all factors in the amount of emissions produced. States produce different amounts of emissions per person due to these factors.
In 2021 , there were 35.3 billion metric tons of energy-related carbon dioxide emissions worldwide.
The Energy Information Administration tracks energy-related greenhouse gas emissions worldwide. While energy is the primary source of emissions, the data is not comprehensive of all greenhouse gas emissions. As of 2021, five countries — China, United States, India, Russia, and Japan — accounted for more than 60% of worldwide energy-related carbon dioxide emissions.
In 2022 , the average temperature in the contiguous US was 1.44°F higher than the 20th-century average of 52.02°F.
Between 1901 to 2000, the average annual temperature of the contiguous us was 52.02°f. so far, every year in the 21st century has been warmer than the 20th-century average..
The National Oceanic and Atmospheric Administration tracks temperature, precipitation and other weather data dating back to 1895. Compiled together, this data illustrates climate trends at various geographic levels, including cities, counties, states, the contiguous US, and the world. Average annual temperatures are calculated by compiling reading from weather stations throughout the year. This data is then compared to a benchmark from a previous average.
While there is weather variation in various times and places, most areas have been warmer in recent decades. Explore Climate in the United States to see how unusual county-level temperatures and precipitation have been in recent years.
In 2022 , 727.7 million acres of land in the US were under protection to maintain wildlife.
The total land protected accounts for 29.8% of all land in the us. that is nearly the same as the three largest states — alaska, texas, and california — combined..
The US Geological Survey (USGS) maintains a database categorizing the 2.4 billion acres of land comprising the United States based on whether it is a protected area. Protected areas are defined by the agency as “dedicated to the preservation of biological diversity and to other natural (including extraction), recreation and cultural uses, managed for these purposes through legal or other effective means.”
Most of that land is owned by the federal government. Four agencies oversee most of the land: Bureau of Land Management, Forest Service, US Fish and Wildlife Service, and National Park Service. The degree of protection varies. Some land protections only apply to endangered and threatened species and can allow for intensive recreation or extraction of natural resources like mining or logging. Others offer complete protection to keep nature undisturbed.
Western states have more land protected than those in the east, both in terms of area and share. Alaska has the most land under protection at over 238 million acres. Nearly 80% of land in Nevada is protected, the highest percentage of any state.
As of December 2023 , there were 939 US-based plant species and 729 US-based animal species listed as either threatened or endangered.
The Fish & Wildlife Service is the federal agency that determines which animal and plant species are considered endangered or threatened in the United States under the Endangered Species Act. Designation grants species federal protections. The agency maintains a current list of all species listed.
In 2015 , Americans were using 990.8 gallons of water per person every day.
Every five years, the US Geological Survey (USGS) releases estimates on how much water is used in the US and how. In 2015, water use was estimated to be 322 billion gallons per day, mostly used for power and irrigation. USGS plans to release 2020 estimates in 2023.
Environment articles
What are the main sources of us greenhouse gas emissions.
In 2021, 7,660 individual facilities were responsible for over 40% of total US emissions.
How many dams does America have?
The average age of a dam in the United States is 61 years, leaving them susceptible to structural failure risks.
What is the most common vehicle fuel type in each state?
Thirty-three million vehicles across the US rely on alternative fuels — which includes more than just electricity.
How dangerous is extreme heat for America’s workers?
Nearly 40 US workers die annually due to environmental heat exposure. Thousands more are injured.
Environment data
SIGN UP FOR THE NEWSLETTER
Keep up with the latest data and most popular content.
- College of Arts & Sciences
- Graduate School of Education & Counseling
Environmental, Natural Resources, & Energy Law Blog
Yinusa saheed olanrewaju - navigating water rights: the complexities of allocation and trust dynamics in the western united states”, view blog here.
Environmental, Natural Resources, and Energy Law is located in Wood Hall on the Law Campus. MSC: 51
email [email protected]
voice 503-768-6649
Environmental, Natural Resources, and Energy Law Lewis & Clark Law School 10101 S. Terwilliger Boulevard MSC 51 Portland OR 97219
- Financial Aid
Why we all need to commit to action for environmental efforts to succeed

The global community is placing more emphasis on waste reduction and a smaller carbon footprint. But what does it really mean to take care of the Earth? Even more, whose responsibility is it?
Indigenous cultures have a longstanding history of celebrating the Earth and fighting to preserve natural resources. While tribes native to America have a complex relationship with the land, protecting our natural resources has always been at the heart of our heritage.
This deep commitment to preservation drives many tribes today to continually work to set the standard for sustainability efforts. For other organizations interested in making positive environmental change, learning from tribes is a good place to start.
For example, Citizen Potawatomi Nation (CPN) launched its own recycling program six years ago to benefit both the environment and the tribe. Each year, the program diverts about 140,000 pounds of recyclable materials from landfills. This allows for perfectly usable materials to be recirculated, in addition to reducing costs to the tribe by decreasing the number of solid waste pickups.
Leadership buy-in is an important key to any organization’s sustainability efforts. This makes investing in environmentally friendly infrastructure, like community gardens, solar panels and other sources of renewable energy, more attainable.
CPN’s administration has shown tremendous support for the tribe’s efforts by making recycling more accessible across its facilities and approving funds to purchase new equipment. Engaged leaders understand what’s good for the planet is also good for the bottom line.
More: Atoka gains a new business, but is 'black mass' it produces at recycling plant safe?
What we have found is that when our leaders champion sustainability efforts, our people are more likely to do the same. On an individual level, tribal members are encouraged to make small changes, which can generate a big impact. This is applicable regardless of tribal affiliation.
First, if you’re not already in the habit of recycling, now is the time to start. If your neighborhood doesn’t offer recycling services, consider making a monthly trip to your local recycling center. Not all materials can be salvaged, though, so research what can be saved and what to throw away.
Additionally, don’t underestimate the power of reusing. Inexpensive single-use items, such as plastic cups and containers, can be replaced with reusable versions that last longer and are more reliable. Reusable shopping bags also continue to grow in popularity, and they help eliminate unnecessary waste during trips to the grocery store. Purchase a few of these bags to keep in the car, and you’ll always be ready for impromptu trips to the store.
For environmental efforts to succeed, we all need to commit to action. This means making positive changes in our personal lives and to our businesses, taking the planet into consideration with each step.
Bryce O’Connor is an environmental specialist in Citizen Potawatomi Nation’s Department of Environmental Protection who oversees the tribe’s recycling program.
Environmental Pollution in the Moscow Region According to Long-term Roshydromet Monitoring Data
- Published: 02 November 2020
- Volume 45 , pages 523–532, ( 2020 )
Cite this article
- G. M. Chernogaeva 1 , 2 ,
- L. R. Zhuravleva 1 ,
- Yu. A. Malevanov 1 ,
- N. A. Fursov 3 ,
- G. V. Pleshakova 3 &
- T. B. Trifilenkova 3
113 Accesses
Explore all metrics
Long-term Roshydromet monitoring data (2009–2018) on the pollution of the atmosphere, soil, and surface water are considered for the Moscow region (Moscow city within its new boundaries and the Moscow oblast). The air quality in the megacity (Moscow) and in background conditions (Prioksko-Terrasny Reserve) is compared.
This is a preview of subscription content, log in via an institution to check access.
Access this article
Price includes VAT (Russian Federation)
Instant access to the full article PDF.
Rent this article via DeepDyve
Institutional subscriptions
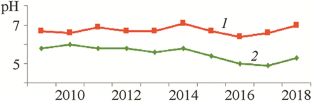
Similar content being viewed by others
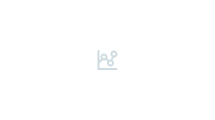
Air Quality in Russian Cities for 1991–2016
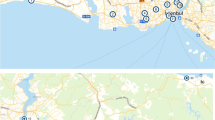
Spatial and temporal look at ten-years air quality of Istanbul city
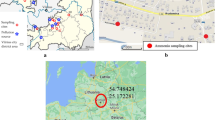
Author information
Authors and affiliations.
Izrael Institute of Global Climate and Ecology, 107258, Moscow, Russia
G. M. Chernogaeva, L. R. Zhuravleva & Yu. A. Malevanov
Institute of Geography, Russian Academy of Sciences, 119017, Moscow, Russia
G. M. Chernogaeva
Central Administration for Hydrometeorology and Environmental Monitoring, 127055, Moscow, Russia
N. A. Fursov, G. V. Pleshakova & T. B. Trifilenkova
You can also search for this author in PubMed Google Scholar
Corresponding author
Correspondence to G. M. Chernogaeva .
Additional information
Russian Text ©The Author(s), 2020, published in Meteorologiya i Gidrologiya, 2020, No. 8, pp. 9-21.
About this article
Chernogaeva, G.M., Zhuravleva, L.R., Malevanov, Y.A. et al. Environmental Pollution in the Moscow Region According to Long-term Roshydromet Monitoring Data . Russ. Meteorol. Hydrol. 45 , 523–532 (2020). https://doi.org/10.3103/S1068373920080014
Download citation
Received : 06 February 2020
Revised : 06 February 2020
Accepted : 06 February 2020
Published : 02 November 2020
Issue Date : August 2020
DOI : https://doi.org/10.3103/S1068373920080014
Share this article
Anyone you share the following link with will be able to read this content:
Sorry, a shareable link is not currently available for this article.
Provided by the Springer Nature SharedIt content-sharing initiative
- Anthropogenic environmental pollution
- Surface water
- Urbanized areas
- Background conditions
- Find a journal
- Publish with us
- Track your research

40 Facts About Elektrostal
Written by Lanette Mayes
Modified & Updated: 10 May 2024

Reviewed by Jessica Corbett
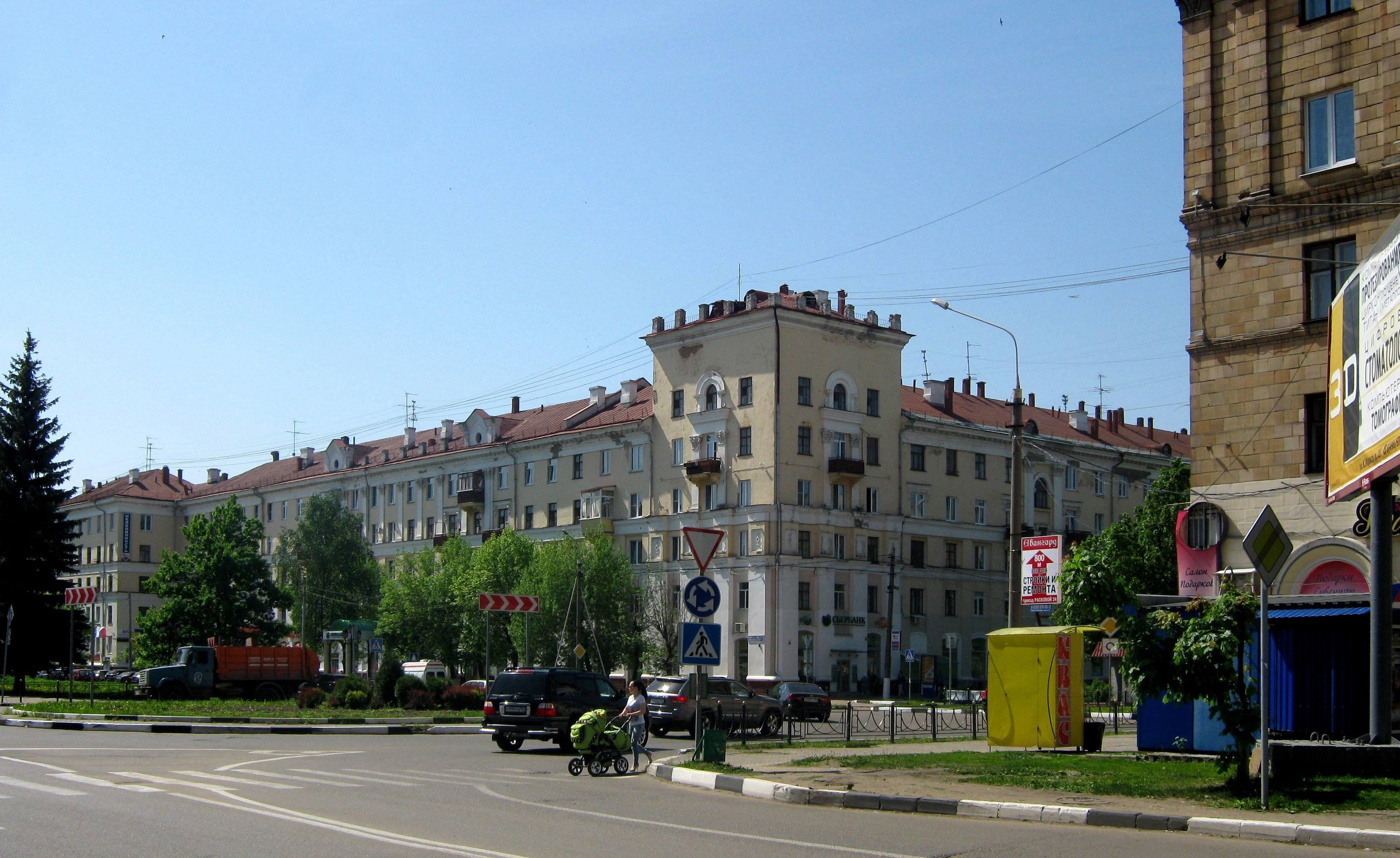
Elektrostal is a vibrant city located in the Moscow Oblast region of Russia. With a rich history, stunning architecture, and a thriving community, Elektrostal is a city that has much to offer. Whether you are a history buff, nature enthusiast, or simply curious about different cultures, Elektrostal is sure to captivate you.
This article will provide you with 40 fascinating facts about Elektrostal, giving you a better understanding of why this city is worth exploring. From its origins as an industrial hub to its modern-day charm, we will delve into the various aspects that make Elektrostal a unique and must-visit destination.
So, join us as we uncover the hidden treasures of Elektrostal and discover what makes this city a true gem in the heart of Russia.
Key Takeaways:
- Elektrostal, known as the “Motor City of Russia,” is a vibrant and growing city with a rich industrial history, offering diverse cultural experiences and a strong commitment to environmental sustainability.
- With its convenient location near Moscow, Elektrostal provides a picturesque landscape, vibrant nightlife, and a range of recreational activities, making it an ideal destination for residents and visitors alike.
Known as the “Motor City of Russia.”
Elektrostal, a city located in the Moscow Oblast region of Russia, earned the nickname “Motor City” due to its significant involvement in the automotive industry.
Home to the Elektrostal Metallurgical Plant.
Elektrostal is renowned for its metallurgical plant, which has been producing high-quality steel and alloys since its establishment in 1916.
Boasts a rich industrial heritage.
Elektrostal has a long history of industrial development, contributing to the growth and progress of the region.
Founded in 1916.
The city of Elektrostal was founded in 1916 as a result of the construction of the Elektrostal Metallurgical Plant.
Located approximately 50 kilometers east of Moscow.
Elektrostal is situated in close proximity to the Russian capital, making it easily accessible for both residents and visitors.
Known for its vibrant cultural scene.
Elektrostal is home to several cultural institutions, including museums, theaters, and art galleries that showcase the city’s rich artistic heritage.
A popular destination for nature lovers.
Surrounded by picturesque landscapes and forests, Elektrostal offers ample opportunities for outdoor activities such as hiking, camping, and birdwatching.
Hosts the annual Elektrostal City Day celebrations.
Every year, Elektrostal organizes festive events and activities to celebrate its founding, bringing together residents and visitors in a spirit of unity and joy.
Has a population of approximately 160,000 people.
Elektrostal is home to a diverse and vibrant community of around 160,000 residents, contributing to its dynamic atmosphere.
Boasts excellent education facilities.
The city is known for its well-established educational institutions, providing quality education to students of all ages.
A center for scientific research and innovation.
Elektrostal serves as an important hub for scientific research, particularly in the fields of metallurgy, materials science, and engineering.
Surrounded by picturesque lakes.
The city is blessed with numerous beautiful lakes, offering scenic views and recreational opportunities for locals and visitors alike.
Well-connected transportation system.
Elektrostal benefits from an efficient transportation network, including highways, railways, and public transportation options, ensuring convenient travel within and beyond the city.
Famous for its traditional Russian cuisine.
Food enthusiasts can indulge in authentic Russian dishes at numerous restaurants and cafes scattered throughout Elektrostal.
Home to notable architectural landmarks.
Elektrostal boasts impressive architecture, including the Church of the Transfiguration of the Lord and the Elektrostal Palace of Culture.
Offers a wide range of recreational facilities.
Residents and visitors can enjoy various recreational activities, such as sports complexes, swimming pools, and fitness centers, enhancing the overall quality of life.
Provides a high standard of healthcare.
Elektrostal is equipped with modern medical facilities, ensuring residents have access to quality healthcare services.
Home to the Elektrostal History Museum.
The Elektrostal History Museum showcases the city’s fascinating past through exhibitions and displays.
A hub for sports enthusiasts.
Elektrostal is passionate about sports, with numerous stadiums, arenas, and sports clubs offering opportunities for athletes and spectators.
Celebrates diverse cultural festivals.
Throughout the year, Elektrostal hosts a variety of cultural festivals, celebrating different ethnicities, traditions, and art forms.
Electric power played a significant role in its early development.
Elektrostal owes its name and initial growth to the establishment of electric power stations and the utilization of electricity in the industrial sector.
Boasts a thriving economy.
The city’s strong industrial base, coupled with its strategic location near Moscow, has contributed to Elektrostal’s prosperous economic status.
Houses the Elektrostal Drama Theater.
The Elektrostal Drama Theater is a cultural centerpiece, attracting theater enthusiasts from far and wide.
Popular destination for winter sports.
Elektrostal’s proximity to ski resorts and winter sport facilities makes it a favorite destination for skiing, snowboarding, and other winter activities.
Promotes environmental sustainability.
Elektrostal prioritizes environmental protection and sustainability, implementing initiatives to reduce pollution and preserve natural resources.
Home to renowned educational institutions.
Elektrostal is known for its prestigious schools and universities, offering a wide range of academic programs to students.
Committed to cultural preservation.
The city values its cultural heritage and takes active steps to preserve and promote traditional customs, crafts, and arts.
Hosts an annual International Film Festival.
The Elektrostal International Film Festival attracts filmmakers and cinema enthusiasts from around the world, showcasing a diverse range of films.
Encourages entrepreneurship and innovation.
Elektrostal supports aspiring entrepreneurs and fosters a culture of innovation, providing opportunities for startups and business development.
Offers a range of housing options.
Elektrostal provides diverse housing options, including apartments, houses, and residential complexes, catering to different lifestyles and budgets.
Home to notable sports teams.
Elektrostal is proud of its sports legacy, with several successful sports teams competing at regional and national levels.
Boasts a vibrant nightlife scene.
Residents and visitors can enjoy a lively nightlife in Elektrostal, with numerous bars, clubs, and entertainment venues.
Promotes cultural exchange and international relations.
Elektrostal actively engages in international partnerships, cultural exchanges, and diplomatic collaborations to foster global connections.
Surrounded by beautiful nature reserves.
Nearby nature reserves, such as the Barybino Forest and Luchinskoye Lake, offer opportunities for nature enthusiasts to explore and appreciate the region’s biodiversity.
Commemorates historical events.
The city pays tribute to significant historical events through memorials, monuments, and exhibitions, ensuring the preservation of collective memory.
Promotes sports and youth development.
Elektrostal invests in sports infrastructure and programs to encourage youth participation, health, and physical fitness.
Hosts annual cultural and artistic festivals.
Throughout the year, Elektrostal celebrates its cultural diversity through festivals dedicated to music, dance, art, and theater.
Provides a picturesque landscape for photography enthusiasts.
The city’s scenic beauty, architectural landmarks, and natural surroundings make it a paradise for photographers.
Connects to Moscow via a direct train line.
The convenient train connection between Elektrostal and Moscow makes commuting between the two cities effortless.
A city with a bright future.
Elektrostal continues to grow and develop, aiming to become a model city in terms of infrastructure, sustainability, and quality of life for its residents.
In conclusion, Elektrostal is a fascinating city with a rich history and a vibrant present. From its origins as a center of steel production to its modern-day status as a hub for education and industry, Elektrostal has plenty to offer both residents and visitors. With its beautiful parks, cultural attractions, and proximity to Moscow, there is no shortage of things to see and do in this dynamic city. Whether you’re interested in exploring its historical landmarks, enjoying outdoor activities, or immersing yourself in the local culture, Elektrostal has something for everyone. So, next time you find yourself in the Moscow region, don’t miss the opportunity to discover the hidden gems of Elektrostal.
Q: What is the population of Elektrostal?
A: As of the latest data, the population of Elektrostal is approximately XXXX.
Q: How far is Elektrostal from Moscow?
A: Elektrostal is located approximately XX kilometers away from Moscow.
Q: Are there any famous landmarks in Elektrostal?
A: Yes, Elektrostal is home to several notable landmarks, including XXXX and XXXX.
Q: What industries are prominent in Elektrostal?
A: Elektrostal is known for its steel production industry and is also a center for engineering and manufacturing.
Q: Are there any universities or educational institutions in Elektrostal?
A: Yes, Elektrostal is home to XXXX University and several other educational institutions.
Q: What are some popular outdoor activities in Elektrostal?
A: Elektrostal offers several outdoor activities, such as hiking, cycling, and picnicking in its beautiful parks.
Q: Is Elektrostal well-connected in terms of transportation?
A: Yes, Elektrostal has good transportation links, including trains and buses, making it easily accessible from nearby cities.
Q: Are there any annual events or festivals in Elektrostal?
A: Yes, Elektrostal hosts various events and festivals throughout the year, including XXXX and XXXX.
Elektrostal's fascinating history, vibrant culture, and promising future make it a city worth exploring. For more captivating facts about cities around the world, discover the unique characteristics that define each city . Uncover the hidden gems of Moscow Oblast through our in-depth look at Kolomna. Lastly, dive into the rich industrial heritage of Teesside, a thriving industrial center with its own story to tell.
Was this page helpful?
Our commitment to delivering trustworthy and engaging content is at the heart of what we do. Each fact on our site is contributed by real users like you, bringing a wealth of diverse insights and information. To ensure the highest standards of accuracy and reliability, our dedicated editors meticulously review each submission. This process guarantees that the facts we share are not only fascinating but also credible. Trust in our commitment to quality and authenticity as you explore and learn with us.
Share this Fact:
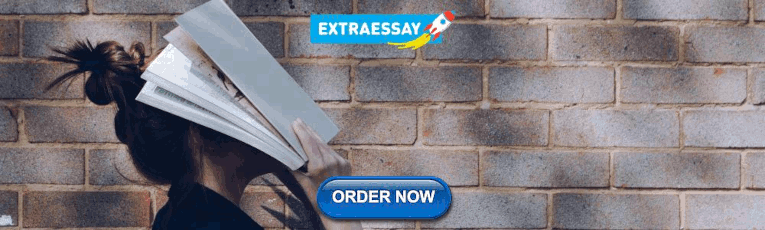
IMAGES
VIDEO
COMMENTS
Natural resource economics focuses on the supply, demand, and allocation of the Earth's natural resources. It's goal is to gain a better understanding of the role of natural resources in the economy. Learning about the role of natural resources allows for the development of more sustainable methods to manage resources and make sure that ...
The use of natural resources has long been considered an element of both human rights and economic development, leading the United Nations, amid its work on advancing decolonization in the 1960s, to declare that "[t]he right of peoples and nations to permanent sovereignty over their natural wealth and resources must be exercised in the interest of their national development and of the well ...
Resources are scarce because we live in a world in which humans' wants are infinite but the land, labor, and capital required to satisfy those wants are limited. This conflict between society's unlimited wants and our limited resources means choices must be made when deciding how to allocate scarce resources.
Global natural resource consumption is forecast to rise 60% by 2060, compared with 2020 levels, according to the United Nations. Increasing demand for resources is due to urbanization, industrialization and a growing population, which is leading to severe consequences such as biodiversity loss, water stress, climate change and air pollution, it says.
Introduction to resource allocation. May 12, 2020. With ongoing habitat loss and degradation, ever-increasing threats to biodiversity, and limited funding for conservation and management, nearly every natural resource manager routinely faces difficult resource allocation problems. Funding and capacity for natural resource management rarely meet ...
These stocks of natural resources, in turn, can be used to produce a flow of goods and services. Each year, we can extract a certain quantity of oil, harvest a certain quantity of fish, and enjoy a certain number of visits to Yellowstone. As with capital, we examine the allocation of natural resources among alternative uses across time.
Natural resource economics deals with the supply, demand, and allocation of the Earth's natural resources. One main objective of natural resource economics is to better understand the role of natural resources in the economy in order to develop more sustainable methods of managing those resources to ensure their availability for future generations.
A central and long-standing question has been how conservation efforts should allocate their limited resources. An important historical point in the United States occurred in the early 1970s when the Nature Conservancy initiated its Natural Heritage Program, a state-level biodiversity database that oriented the conservation targets away from ...
The extraction of natural resources is essential for both developed and developing economies for economic growth and sustainable usage; natural resource rents also pave the way (He et al., 2022; Shahbaz et al., 2018) Fig. 2. Represents the natural gas volatility from 1984 to 2021 and the severe downfall during Covid-19 and the Russian-Ukrainian ...
The failure of the market to allocate natural resources efficiently due to the externality and public good characteristics problem creates the necessity of economic measures of values to guide policymaking (Freeman III et al., 2014). A significant concern, thus, arises in determining how nature or the environment should be valued.
In turn, if μ reflects the portion in society's basket of goods allocated to consumption of the natural resource, then our definition is consistent with the way the marginal productivity of ...
Different regions have access to different renewable or nonrenewable natural resources such as freshwater, fossil fuels, fertile soil, or timber based on their geographic location and past geologic processes. Access, or the lack thereof, contributes to a place's economic development, political relationships, and culture. For example, the Great Plains region of the United States is known for ...
Allocating natural resource reduction amounts: A data envelopment analysis based-approach considering production technology heterogeneity. Jiayun Song, ... Previous studies resource allocation methods based on data envelopment analysis assume that all the assessed decision-making units share a common production technology, and all decision ...
Real Costs of Natural Resource Exploitation. Ernie Jowsey. Environmental Science, Economics. 2006. Natural resources are usually termed either renewable or non-renewable. Renewable resources include 'biological' resources, such as fish and forests and also energy flow resources such as hydro,…. Expand. 3. 1 Excerpt.
The Supreme Court first observed that auction was the best way to allocate natural resources, be it oil and gas, spectrum, minerals and coal. However, later in September, 2012, the Supreme Court clarified that it does not believe that all national resources must be auctioned. In fact, it opined auction cannot be the sole criteria for alienation ...
A natural resource is anything found in nature that can be used by humans. There are two types of natural resources: renewable and nonrenewable. Renewable resources can be replaced over human lifetimes. Nonrenewable resources cannot. Renewable resources are unevenly distributed around Earth.
Natural resources are materials from the earth that people use to meet their needs. The United States has an unusual abundance of six natural resources: a large landmass, two expansive coastlines, a wide swath of fertile land, abundant fresh water, huge reserves of oil and coal, and a diverse population.
In 2020, a net total of 5.2 billion metric tons of carbon dioxide (or equivalent) was emitted in the US. That's 15.8 metric tons per person. Find statistics and data trends about the environment, including data on the weather that helps interpret climate trends, greenhouse gas emissions caused by human activity, and conservation-related metrics such as land protections and species listed on ...
Department of Environmnental Medicine, Palo Alto Medical Clinic, Palo Alto, California 94301
Environmental, Natural Resources, & Energy Law Blog Yinusa Saheed Olanrewaju - Navigating Water Rights: The Complexities of Allocation and Trust Dynamics in the Western United States"
While tribes native to America have a complex relationship with the land, protecting our natural resources has always been at the heart of our heritage. This deep commitment to preservation drives many tribes today to continually work to set the standard for sustainability efforts. For other organizations interested in making positive ...
Report on Natural Resources and Environmental Conditions in the Moscow Oblast in 2018. Information Bulletin (Ministry of Natural Resources and the Environment of the Moscow Oblast, 2019) [in Russian]. Soil Contamination in the Russian Federation with Industrial Toxic Substances. Yearbook (NPO Taifun, Obninsk, 2009-2018) [in Russian].
Moscow Oblast (Russian: Московская область, romanized: Moskovskaya oblast, IPA: [mɐˈskofskəjə ˈobləsʲtʲ], informally known as Подмосковье, Podmoskovye, IPA: [pədmɐˈskovʲjə]) is a federal subject of Russia (an oblast).With a population of 8,524,665 (2021 Census) living in an area of 44,300 square kilometers (17,100 sq mi), it is one of the most densely ...
2.1. Problem description. The problem of fibre allocation in optical cable production can be described as follows: in the scheduling task, there are bundle number set N, the length set L, the number of tubes K, the number of fibres P, L i is the length of product i, N i is the number of bundles of product i, each length l corresponds to a bundle number n, that is, the elements of the bundle ...
40 Facts About Elektrostal. Elektrostal is a vibrant city located in the Moscow Oblast region of Russia. With a rich history, stunning architecture, and a thriving community, Elektrostal is a city that has much to offer. Whether you are a history buff, nature enthusiast, or simply curious about different cultures, Elektrostal is sure to ...
Main page; Contents; Current events; Random article; About Wikipedia; Contact us; Donate; Pages for logged out editors learn more