Thank you for visiting nature.com. You are using a browser version with limited support for CSS. To obtain the best experience, we recommend you use a more up to date browser (or turn off compatibility mode in Internet Explorer). In the meantime, to ensure continued support, we are displaying the site without styles and JavaScript.
- View all journals
- My Account Login
- Explore content
- About the journal
- Publish with us
- Sign up for alerts
- Open access
- Published: 08 June 2020
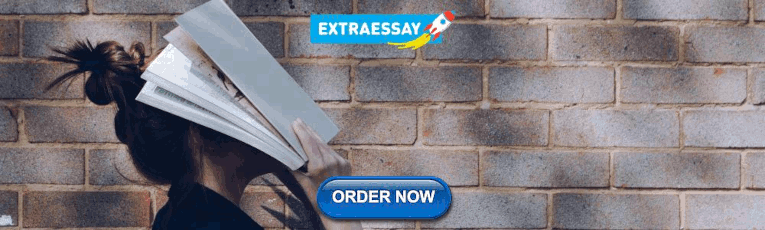
Bioremediation of oil contaminated soil using agricultural wastes via microbial consortium
- Chao Zhang 1 , 2 ,
- Daoji Wu 1 , 2 &
- Huixue Ren 1 , 2
Scientific Reports volume 10 , Article number: 9188 ( 2020 ) Cite this article
33 Citations
1 Altmetric
Metrics details
- Environmental biotechnology
- Soil microbiology
Agricultural wastes, such as wheat bran and swine wastewater, were used for bioremediation of oil-contaminated soil. Two optimised strains that could degrade oil efficiently were selected. The result showed that the best ratio of strain A to strain B was 7:3. Swine wastewater could be a replacement for nitrogen source and process water for bioremediation. Next, the Box-Behnken design was used to optimise the culture medium, and the optimal medium was as follows: microbial dosage of 97 mL/kg, wheat bran of 158 g/kg and swine wastewater of 232 mL/kg. Under the optimal medium, the oil degradation rate reached 68.27 ± 0.71% after 40 d. The urease, catalase, and dehydrogenase activities in oil-contaminated soil all increased, and the microbe quantity increased significantly with manual composting. These investigations might lay a foundation for reducing the pollution of agricultural wastes, exploring a late model for bioremediation of oil-contaminated soil.
Similar content being viewed by others
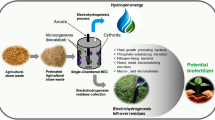
Using bioelectrohydrogenesis left-over residues as a future potential fertilizer for soil amendment
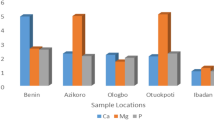
Bio-augmentation and bio-stimulation with kenaf core enhanced bacterial enzyme activities during bio-degradation of petroleum hydrocarbon in polluted soil
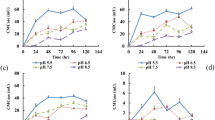
Enhancing the compost maturation of swine manure and rice straw by applying bioaugmentation
Introduction.
A large number of oil pollutants are produced during the production, processing, transportation, and utilisation of oil 1 , 2 . This has caused serious soil pollution. Therefore, it is urgent to repair the oil-contaminated soil. Compared with physical repair and chemical repair, bioremediation has been widely used because of its advantages of good effect, easy operation, low cost, rapid degradation rate, and lack of secondary pollution 3 , 4 .
Bioremediation is divided into two types of remediation: in-situ and ex-situ . In-situ remediation techniques include land tillage, microorganism addition, bio-culture, and bio-ventilation. Ex-situ remediation technologies include prefabricated bed and bioreactor 5 , 6 . For example, Besalatpour et al 7 . used land tillage to remediate 500 kg of oil-contaminated soil in farmland. After four months, total petroleum hydrocarbons decreased by 50%. The essence of bioremediation technology is the degradation of pollutants through microbial metabolic activities. A majority of the microorganisms used in the process of remediation are indigenous microorganisms 8 . In order to improve the repair effect, domesticated high-efficiency oil-degrading bacteria were introduced. Wu et al 9 . repaired the contaminated soil with 82,533 mg/kg petroleum hydrocarbon content by adding mixed degrading bacteria. After 13 weeks, the content of petroleum hydrocarbon decreased to 47,600 mg/kg, and the removal rate of petroleum hydrocarbon reached 42.3%. Although there are many scholars engaged in this field, there remains a lack of low-cost and positive-effect technology. Existing investigations reported that the expensive refined supplements were still necessary for bioremediation, such as refined carbon source, refined nitrogen source, process water, or surface active agent. Therefore, the high cost is the bottleneck of bioremediation.
Swine wastewater mainly includes pig urine, partial pig manure, and piggery flushing water. These types of wastewater include high concentrations of organic matter (biochemical oxygen demand 4.5-8.0 g/L), ammonia nitrogen (1.3-1.5 g/L), and total nitrogen (1.6-2.2 g/L) 10 . It is a kind of organic wastewater which is difficult to treat. If discharged directly into local bodies of water, it will cause enormous environmental pollution. Dealing with the discharge according to standards is very difficult and costly. If the wastewater can be used as a medium for remediation of contaminated soil, not only can the cost of remediation be greatly reduced but also the wastewater can be recycled, and the pollution to the environment can be reduced.
Two strains ( Bacillus subtilis CICC 21312 and Candida bombicola ATCC 22214) had good oil decomposing ability. Accordingly, they were used for bioremediation of simulated oil-contaminated soil in the present study. Swine wastewater was used instead of a nitrogen source and process water for bioremediation. The optimal ratio of strains and the optimal medium for mixed strains were determined. In addition, the soil changes after bioremediation were studied to understand physicochemical properties, enzyme activities, and microbial population, which provided technical reference and theoretical support for the field application of bioremediation in DongYing oil-contaminated soil.
Comparison of oil degradation rates among different strains and indigenous microorganisms
The main component of petroleum pollutants in soil is polycyclic aromatic hydrocarbons (PAHs), yet the solubility of PAHs in soil aqueous solution is very low, and PAHs can be adsorbed on soil particles, resulting in poor bioavailability. This retards the biodegradation rate of PAHs at the solid-liquid interface of soil 11 . Surfactant is a kind of macromolecule with both hydrophilic and hydrophobic groups. It can increase the contact area between PAHs and soil microorganisms, improving the availability and degradation rate by curling, solubilisation, and elution 12 . However, exogenous surfactants increase the cost of soil remediation. Therefore, a strain producing sophorolipid(strain B) was selected as the soil remediation strain in this study. At the same time, sophorolipid is degradable and does not easily cause secondary pollution.
In order to select better oil degrading bacteria, four strains were selected for comparison. The comparison of oil degradation rates among different strains and indigenous microorganisms is shown in Fig. 1 . The results shows that A and B have high oil degradation rate. The oil degradation rate of C and D is relatively low, so no further study is required. Because the effects of A and B are high, the synergistic effect of A and B is also investigated. The sequence of oil degradation rate of strains is A + B > A (30.08 ± 1.08%)> B (21.19 ± 1.30%), which are higher than indigenous microorganisms. After 40 d culture of strain A + B, the oil degradation rate was 41.12 ± 1.50%, while the oil degradation rate of indigenous microorganisms after 40 d was only 5.02 ± 0.15%. The main reason for the treatment effect of the mixed bacteria being better than that of the single bacterium was that strain B was a sophorolipid-producing bacteria, which could degrade PAHs well. Moreover, there was no competition between strain B and strain A, which could be mixed cultured. Strain A degraded alkane, and strain B degraded PAHs. They played a complementary role, so the degradation rate reached the optimal value.
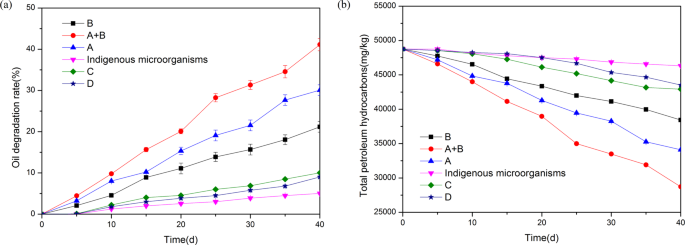
Comparison of oil degradation rates among different strains and indigenous microorganisms (▲: A; ▼ : Indigenous microorganisms; ● : A + B; ■: B; ♦: C; ★ : D); ( a ) Oil degradation rate (%); ( b ) Total petroleum hydrocarbons (mg/kg).
Construction of dominant flora and determination of the optimal ratio
The effect of mixed proportions (A:B) on oil degradation rate were investigated; the results are shown in Fig. 2 .
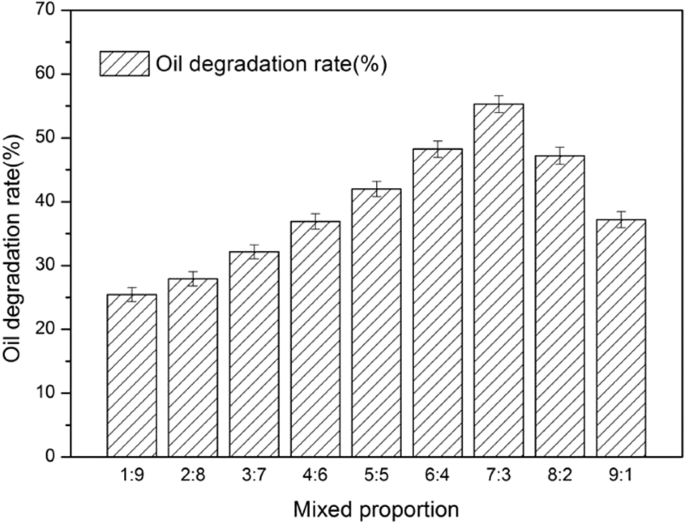
Effect of mixed proportion (A,B) on the oil degradation rate.
As can be seen, the proportion of strains affected the oil degradation rate. A ratio that was too low or too high reduced the oil degradation rate. The maximum oil degradation rate was 55.31 ± 1.32% at 7:3.
Box-Behnken design and response surface analysis
The best level of the three factors (inoculation amount of mixed strains, the amount of wheat bran and the amount of swine wastewater) was determined by BBD. The factor levels used in the BBD are shown in Table 1 , and results are in Table 2 . Variance for the quadratic design is in Table 3 .
Values of “Prob> F” less than 0.0500 indicate model terms are significant. In this case F, F 2 , and G 2 are significant model terms. The final coding factor formula is as follows:
Oil degradation rate = 65.19 – 1.79 × E + 3.98 × F + 1.04 × G – 0.078 × E × F + 1.68 × E × G + 0.56 × F × G – 2.45×E 2 – 5.14 × F 2 – 4.44 × G 2 (1) where Y is oil degradation rate, E is the inoculation amount, F is the amount of wheat bran, and G is the amount of swine wastewater.
F test was used to judge the significance of each variable in the regression equation to the response value. The smaller the probability of P, the higher the corresponding variable. The first order term F (P < 0.05) of model (1) was significant, while E and G (P > 0.05) had no significant effects. The effects of secondary order item F 2 , G 2 (P < 0.05) was significant, and E 2 (P > 0.05) had no significant effects. There was no significant difference between EF, EG, and FG (P > 0.05).
These results clearly showed that experimental values had a linear distribution and a good correlation (R 2 = 0.9912). Therefore, the model could be used to predict the degradation rate of crude oil in the variable range. The maximum oil degradation rate of 68.22% was obtained at 96.53 mL/kg inoculation, 157.85 g/kg wheat bran and 232.29 mL/kg swine wastewater. To validate this prediction, three independent experiments were carried out, and an oil degradation rate of 68.27 ± 0.71% was obtained via 97 mL/kg inoculation, 158 g/kg wheat bran and 232 mL/kg swine wastewater.
The effects of the inoculation amount of mixed strains, amount of wheat bran and amount of swine wastewater on oil degradation rate were analysed by RSM. As shown in Fig. 3 , three-dimensional response surface plots and contour plots were generated. This allows for studying the interaction of any two variables on the response. From the observation of the response surface graph of the interaction between the two factors, there was a strong interaction among the factors, and it was found that the steeper the curve trend, the more significant the influence. The smoother the curve trend, the smaller the influence. The shape of the contour plot represents the strength of interaction. The contour plot was round, indicating that the interaction between the two factors was weak. Moreoeer, the contour plot was oval, indicating that the interaction between the two factors was strong. Comparing the highest points and contours of the response surface in Fig. 3 , there were extreme values in the selected range.
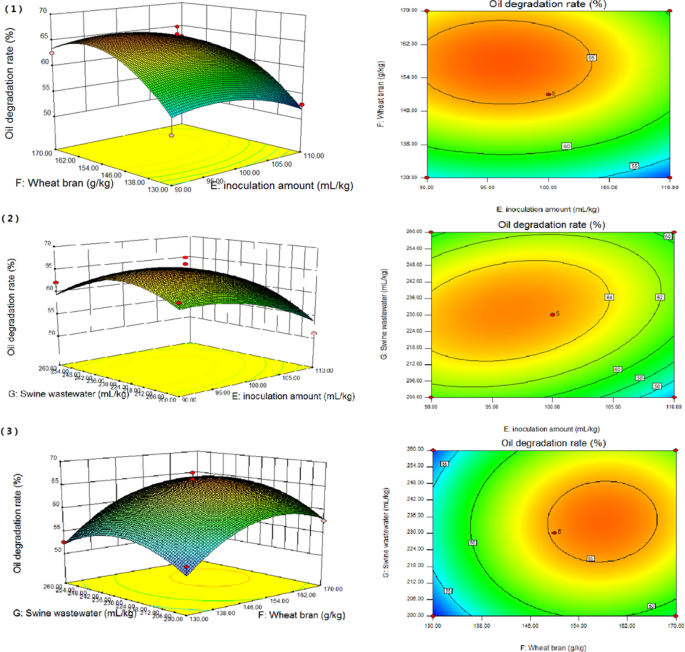
Surface and contour plots of mutual-influence. (1) effect of inoculation amount ( E ) and wheat bran ( F ); (2) effect of inoculation amount ( E ) and swine wastewater ( G ); and (3) effect of wheat bran ( F ) and swine wastewater ( G ).
Comparison of soil properties before and after bioremediation
Oil-contaminated soil, oil contaminated soil treated by indigenous microbial aeration composting, and oil-contaminated soil treated by microbial consortium (optimal ratio and optimal culture conditions) were recorded as M1, M2, M3, respectively. The changes of the soil’s physical and chemical properties, enzyme activities, and microbial population before and after bioremediation were investigated. The results are shown in Table 4 .
After comparing three kinds of soil samples, M3 had the best recovery effect, and the oil degradation rate reached 68.27 ± 0.71%. The oil degradation rate of M2 was only 5.02 ± 0.27%. Large amounts of nutrients were put into the sample in the process of M3 repair, so the content of organics and total nitrogen in the soil were greatly improved, while the changes in the corresponding content in M2 were not as obvious as that in M3.
The activities of dehydrogenase and catalase in M3 were the highest. This was due to the large amount of soil microorganism reproduction during the repair process, and the microorganism participated in the degradation process of oil hydrocarbon, which improved the activity of dehydrogenase and catalase. The urease activity in M3 was the highest, indicating that the soil had a strong nitrogen conversion ability, which was beneficial to the growth of microorganisms in the soil. It also provided sufficient nutritional conditions for the microorganism to participate in the degradation of oil hydrocarbon. However, the activities of various enzymes in M2 were not as obvious as those in M3.
Bacteria counts, actinomycetes counts, and fungi counts in M3 were significantly higher than that of untreated soil sample M1 and soil sample M2 after ordinary composting, which were close to the actual microbial number in the natural world.
The remediation of crude oil contaminated soil is a worldwide problem. Although there exist many remediation methods, this technology is still a promising remediation method. The comparison of different method was shown in Table 5 . The order of oil degradation rate of different methods saw this study as better than Besalatpour et al. which was better than Wu et al. In this study, the remediation time was the shortest, and the problem of piggery wastewater pollution was solved. Moreover, it had the following advantages: (1) The treatment cost was low. In the process of treatment, all materials except strains were wastes. (2) The process could offset the disposal costs of agricultural wastes. (3) No exogenous chemical surfactants were needed during the remediation process, because strain B produced sophorolipids. (4) Swine wastewater could be a replacement for process water in bioremediation.
The main reason for the lack of consideration of the effect of aeration on microflora was as follows: although many microorganisms were aerobic, they could not be supplied with oxygen in the remediation of oil-contaminated soils. It increased the processing cost to an unaffordable level. Two highly-efficient oil-degrading strains preserved in the laboratory could improve the effect of bioremediation of oil contaminated soil. The recovery efficiency of single strain in 40 d repair process was much higher than that of indigenous microorganisms. The order of oil degradation rate of two strains was A + B > A > B. The combination of A and B had the best oil degradation rate. The best ratio (mass ratio) of the two strains was 7:3. Under this condition, the oil degradation rate of soil reached 55.31 ± 1.32%. Swine wastewater could be a replacement for nitrogen source and process water for bioremediation. The optimal medium was microbial dosage of 97 mL/kg, wheat bran of 158 g/kg and swine wastewater of 232 mL/kg. Under the optimal medium, the oil degradation rate reached 68.27 ± 0.71% after 40 d. The fertility of oil-contaminated soil, which was repaired by microbial consortium, increased significantly. In addition, the activities of dehydrogenase, peroxidase, and urease increased, and the number of microbes increased significantly. These investigations may lay a foundation for reducing the pollution of agricultural wastes, exploring a late model for bioremediation of oil-contaminated soil. In the future experiments, this microbial consortium will be studied to repair the oil-contaminated soil in other places.
Materials and Methods
Soil samples.
Crude oil was obtained from DongYing refinery, China. The characteristic parameters of the crude oil were 18.5% polycyclic aromatic hydrocarbon and 48.4% alkane. Soil samples were collected from the unpolluted shallow (5~25 cm) soil in DongYing, China. Soil samples were broken, cleaned, mixed and sieved. According to the ratio of crude oil to soil mass ratio (1:20), the oil contaminated soil was made ready for use. Next, soil was sealed in the sterilised kraft paper bag, maintained in cold storage 13 .
Swine wastewater
The swine wastewater used in this study was effluent coming from ZhengBang pig farm in DongYing, China. The characteristic parameters of the swine wastewater were as follows: 15.4 ± 0.2 g/L chemical oxygen demand (COD Cr ), 6.5 ± 0.2 g/L biochemical oxygen demand (BOD 5 ), 883 ± 12 mg/L suspended solid (SS), 1.1 ± 0.1 g/L ammonia nitrogen (NH 3 -N), 1.8 ± 0.1 g/L total nitrogen, 40.5 ± 0.5 mg/L total phosphorus, and pH of 7.5 ± 0.2. The wastewater was autoclaved for 20 min at 121 °C.
Bacillus subtilis X-12(A) was purchased from the China Center of Industrial Culture Collection (CICC) as CICC 21312. Candida bombicola C-15(B) was purchased from the American Type Culture Collection (ATCC) as ATCC 22214. Strains were maintained on LB medium or YPD medium at 4 °C. Pseudomonas aeruginosa A-21(C) was purchased from the CICC as CICC 10204. Arthrobacter sp .D-2 (D) was purchased from the CICC as CICC 10758.
LB medium: yeast extract, 5 g/L; peptone, 10 g/L; sodium chloride, 5 g/L; and agar 18 g/L.
YPD medium: yeast extract, 10 g/L; peptone, 20 g/L; glucose, 20 g/L; and agar 18 g/L.
Seed medium: yeast extract, 10 g/L; peptone, 20 g/L; and glucose, 20 g/L.
All media were autoclaved for 20 min at 121 °C.
Comparison of oil degradation rates between two strains and indigenous microorganisms
Two strains were actived in seed medium. The concentration of microbial cells was 1.5×10 8 CFU/mL. The oil contaminated soil was packed into 48 conical bottles (50 g each). The conical flasks were divided into three groups, with 16 flasks in each group. Then, 50 mL/kg of strain A and strain B suspension were added to two groups, and put in the incubator (28 °C). They were watered and ploughed every day to keep the water content around 20%. The remaining group (control group) did not receive strain suspension for investigating the effect of indigenous microorganisms. Samples were taken at intervals, and the oil degradation rate was calculatedby gravimetric method to checkthe mass fraction of oil in soil samples 14 .
Combinations of two strains were carried out. The mixed bacteria of different combinations were added into the oil-contaminated soil according to a certain amount of inoculation, and the total inoculation amount (50 mL/kg) of the mixed bacteria suspension in each group was equal. They were watered and ploughed every day to keep the water content around 20%. After 40 days in the incubator (28 °C), the mass fraction of oil in the soil samples was determined, and the oil degradation rate was calculated.
Three factors affecting the oil degradation rate (inoculation amount of mixed strains, the amount of wheat bran and the amount of swine wastewater) were selected. Box-Behnken design (BBD) was used to determine optimal concentrations of factors using Design-Expert software (Version 8.0.6, Stat-Ease, Inc, USA), and to understand the relationship among various factors. The three factors were studied at three levels (Table 1 ), and 17 sets of experiments were carried out (Table 2 ). All experiments were carried out in triplicate. The optimal values of factors were obtained by analysing 3D plots. The statistical analysis of the model was represented as an analysis of variance (ANOVA).
Analytical methods
The pH of soil samples was measured by potentiometric titration, and the volume ratio of water to soil was 2.5. The content of organic matter in soil samples was determined by potassium dichromate volumetric method. The total nitrogen content of the soil sample was determined by the semi-micro kjeldahl method. The content of total phosphorus in a soil sample was determined by the Mo-Sb antispetrophotography method 15 .
The total petroleum hydrocarbons (TPH) content in soil was determined by the gravimetric method 14 . The calculation formula for the oil degradation rate was as follows:
where ODR was the oil degradation rate (%), W 1 was the content of petroleum hydrocarbon in the soil before degradation, and W 2 was the content of petroleum hydrocarbon in the soil after degradation.
The amount of polycyclic aromatic hydrocarbon and alkane in soil were measured by the gravimetric method 14 .
Total nitrogen, total phosphorus, suspended solid, NH 3 -N, BOD 5 , and COD Cr in the swine wastewater were measured according to the procedure described in standard methods for the examination of water and wastewater 15 .
The activity of dehydrogenase was determined by using triphenyltetrazolium chloride as hydrogen acceptor, which was reduced to form red formazan. It was determined by colorimetry. The amount of formazan produced in 1 g of dry soil for 6 h was an active unit of dehydrogenase. The urease activity in the soil sample was determined by Nessler’s reagent colorimetry. The amount of NH 3 -N produced in 24 h in 1 g of dry soil was an activity unit of urease. Polyphenol oxidase activity in a soil sample was determined by pyrogallol colorimetry. The amount of gallic acid produced in 3 h in 1 g of dry soil was taken as an activity unit of polyphenol oxidase. Catalase activity in a soil sample was determined by Potassium Permanganate titration. The enzyme activity was expressed by the volume number of potassium permanganate consumed in 1 g dry soil in 1h 16 . The amount of microbial population in the soil was determined by colony forming unit 17 .
Das, N. & Chandran, P. Microbial Degradation of Petroleum Hydrocarbon Contaminants: An Overview. Biotechnology Research International,2011,(2010-07-27) 2011 , 941810 (2010).
Google Scholar
Erdogan, E. & Karaca, A. Bioremediation of Crude Oil Polluted Soils . Vol. 3 (2011).
Agamuthu, P., Tan, Y. S. & Fauziah, S. H. Bioremediation of Hydrocarbon Contaminated Soil Using Selected Organic Wastes ☆ . Procedia Environmental Sciences 18 , 694–702 (2013).
Article CAS Google Scholar
Hesnawi, R. M. & Mogadami, F. S. Bioremediation of Libyan Crude Oil-Contaminated Soil under Mesophilic and Thermophilic Conditions ☆ . Apcbee Procedia 5 , 82–87 (2013).
Liu, Q., Tang, J., Bai, Z., Hecker, M. & Giesy, J. P. Distribution of petroleum degrading genes and factor analysis of petroleum contaminated soil from the Dagang Oilfield, China. Scientific Reports 5 , 11068 (2015).
Article ADS CAS Google Scholar
Streche, C., Cocârţă, D. M., Istrate, I. A. & Badea, A. A. Decontamination of Petroleum-Contaminated Soils Using The Electrochemical Technique: Remediation Degree and Energy Consumption. Scientific Reports 8 , 3272 (2018).
Article ADS Google Scholar
Besalatpour, A., Hajabbasi, M. A., Khoshgoftarmanesh, A. H. & Dorostkar, V. Landfarming Process Effects on Biochemical Properties of Petroleum-Contaminated Soils. Journal of Soil Contamination 20 , 15 (2011).
Banks, M. K. et al . The effect of plants on the degradation and toxicity of petroleum contaminants in soil: a field assessment. Advances in Biochemical Engineering/biotechnology 78 , 75 (2003).
Wu, M. et al . Bioremediation of petroleum contaminated soil and activity of hydrocarbon ediation of petroleum contaminated soil and activity of hydrocarbon degrading bacteria. Chinese Journal of Applied and Environmental Biology 22 , 0878-0883 (2016).(in chinese)
Cheng, D. L. et al . Bioprocessing for elimination antibiotics and hormones from swine wastewater. Science of the Total Environment 621 , S0048969717327626 (2018).
Article Google Scholar
Huang, H. et al . The naphthalene catabolic protein NahG plays a key role in hexavalent chromium reduction in Pseudomonas brassicacearum LZ-4. Scientific Reports 7 , 9670 (2017).
Kachienga, L., Jitendra, K. & Momba, M. Metagenomic profiling for assessing microbial diversity and microbial adaptation to degradation of hydrocarbons in two South African petroleum-contaminated water aquifers. Scientific Reports 8 , 7564 (2018).
Sarkar, D., Ferguson, M., Datta, R. & Birnbaum, S. Bioremediation of petroleum hydrocarbons in contaminated soils: comparison of biosolids addition, carbon supplementation, and monitored natural attenuation. Environmental Pollution 136 , 187–195 (2005).
Xu, R. & Obbard, J. P. Effect of nutrient amendments on indigenous hydrocarbon biodegradation in oil-contaminated beach sediments. Journal of Environmental Quality 32 , 1234 (2003).
Eaton, A. D., Greenberg, A. E., Clesceri, L. S. & Franson, M. A. H. Standard Methods for The Examination of Water & Wastewater . (1995).
Dindar, E., Şağban, F. O. T. & Başkaya, H. S. Variations of soil enzyme activities in petroleum-hydrocarbon contaminated soil. International Biodeterioration & Biodegradation 105 , 268–275 (2015).
Yeung, P. Y., Johnson, R. L. & Xu, J. G. Biodegradation of Petroleum Hydrocarbons in Soil as Affected by Heating and Forced Aeration. Journal of Environmental Quality 26 , 1511–1516 (1997).
Download references
Acknowledgements
This research was financially supported by State Key Laboratory of Microbial Technology (M2012-14), Shandong University.
Author information
Authors and affiliations.
School of Municipal and Environmental Engineering, Shandong Jianzhu University, JiNan, 250101, China
Chao Zhang, Daoji Wu & Huixue Ren
Co-Innovation Center of Green Building, JiNan, 250101, China
You can also search for this author in PubMed Google Scholar
Contributions
C.Z. and D.J.W. conceived of the study. C.Z. designed experiments, analyzed data, and performed experiments with assistance from H.X.R. C.Z. drafted the manuscript. All authors read and approved the final manuscript.
Corresponding author
Correspondence to Daoji Wu .
Ethics declarations
Competing interests.
The authors declare no competing interests.
Additional information
Publisher’s note Springer Nature remains neutral with regard to jurisdictional claims in published maps and institutional affiliations.
Rights and permissions
Open Access This article is licensed under a Creative Commons Attribution 4.0 International License, which permits use, sharing, adaptation, distribution and reproduction in any medium or format, as long as you give appropriate credit to the original author(s) and the source, provide a link to the Creative Commons license, and indicate if changes were made. The images or other third party material in this article are included in the article’s Creative Commons license, unless indicated otherwise in a credit line to the material. If material is not included in the article’s Creative Commons license and your intended use is not permitted by statutory regulation or exceeds the permitted use, you will need to obtain permission directly from the copyright holder. To view a copy of this license, visit http://creativecommons.org/licenses/by/4.0/ .
Reprints and permissions
About this article
Cite this article.
Zhang, C., Wu, D. & Ren, H. Bioremediation of oil contaminated soil using agricultural wastes via microbial consortium. Sci Rep 10 , 9188 (2020). https://doi.org/10.1038/s41598-020-66169-5
Download citation
Received : 17 September 2019
Accepted : 13 May 2020
Published : 08 June 2020
DOI : https://doi.org/10.1038/s41598-020-66169-5
Share this article
Anyone you share the following link with will be able to read this content:
Sorry, a shareable link is not currently available for this article.
Provided by the Springer Nature SharedIt content-sharing initiative
This article is cited by
Comparative genomics analysis of the aromatic and xenobiotic degradation capacities and heavy metal resistance in seven environmentally derived bacterial isolates.
- Alexander R. Kneubehl
Water, Air, & Soil Pollution (2023)
Acidophilic microorganisms in remediation of contaminants present in extremely acidic conditions
- Sultana Razia
- Tony Hadibarata
- Sie Yon Lau
Bioprocess and Biosystems Engineering (2023)
Advanced bioremediation by an amalgamation of nanotechnology and modern artificial intelligence for efficient restoration of crude petroleum oil-contaminated sites: a prospective study
- Rupshikha Patowary
- Arundhuti Devi
- Ashis K. Mukherjee
Environmental Science and Pollution Research (2023)
By submitting a comment you agree to abide by our Terms and Community Guidelines . If you find something abusive or that does not comply with our terms or guidelines please flag it as inappropriate.
Quick links
- Explore articles by subject
- Guide to authors
- Editorial policies
Sign up for the Nature Briefing: Translational Research newsletter — top stories in biotechnology, drug discovery and pharma.

Bioremediation of Oil Spills: A Review of Challenges for Research Advancement
- Babajide Milton Macaulay University of Greenwich
- Deborah Rees University of Greenwich
As the demand for liquid petroleum increases, the need for reliable and efficient oil spill clean-up techniques is inevitable. Bioremediation is considered one of the most sustainable clean-up techniques but the potential has not been fully exploited in the field because it is too slow to meet the immediate demands of the environment. This study reviews the challenges to managing oil spills in terrestrial and marine environments to identify areas that require further research. Current challenges associated with bioremediation of spilled petroleum include resistance of asphalthenes to biodegradation; delay of heavy or high molar mass polycyclic aromatic hydrocarbon (PAH) biodegradation, eutrophication caused by biostimulation, unsustainability of bioaugmentation in the field, poor bioavailability of spilled petroleum, inefficiency of biodegradation in anoxic environments and failure of successful bioremediation laboratory studies in the field. Recommendations offered include encouraging asphalthene biodegradation by combining heat application (80°C), biosurfactant (thermophilic emulsifier) and bioaugmentation (using a consortium containing Bacillus lentus and Pleurotus tuberregium as members) but as a temporary measure, adopting the use of "booms and skimmers" and "organic sorbents" for water and land clean-up, respectively. Heavy PAHs may be rapidly degraded by applying nutrients (biostimulation) and biosurfactants to sites that are oleophilic microbe-rich. Oleophilic nutrients may be the most effective strategy to reduce eutrophication in marine environments whilst on land, slow-release nutrient application or organic-inorganic nutrient rotation may help prevent soil hardening and infertility. The use of encapsulating agents and genetically-engineered microbes (GEMs) may increase the efficiency of bioaugmentation in the field, but temporarily, indigenous oleophilic microbes may be employed in the field. Poor bioavailability of crude oil may be eliminated by the use of biosurfactants. In terrestrial anoxic sites, bioslurping-biosparging technology could be used whilst the marine anoxic site requires more research on how to transport nutrients and biosurfactants to oleophilic anaerobes residing in the ocean beds. The involvement of both governmental and non-governmental environmental institutions in sponsoring field studies in order to improve the reliability of bioremediation research. Further studies to test the practicability and cost of these recommendations in the field are needed.
Permanent URL: http://hdl.handle.net/2047/d20018675
How to Cite
- Endnote/Zotero/Mendeley (RIS)
Information
- For Readers
- For Authors
- For Librarians
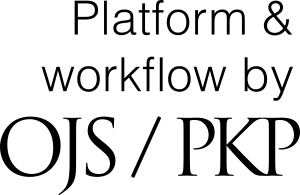
American Society for Microbiology
How microbes clean up oil: lessons from the deepwater horizon oil spill.
April 19, 2020
How Do Microbes Respond to Oil Spills?
How do microbes respond to cleanup efforts, what happens to oil that reaches the seafloor, what does the future hold for the gulf.
- Nutrient Cycling
- Bioremediation
Author: Jennifer Tsang, Ph.D.

ASM Microbe 2024 Registration Now Open!
Discover asm membership, get published in an asm journal.
Academia.edu no longer supports Internet Explorer.
To browse Academia.edu and the wider internet faster and more securely, please take a few seconds to upgrade your browser .
Enter the email address you signed up with and we'll email you a reset link.
- We're Hiring!
- Help Center
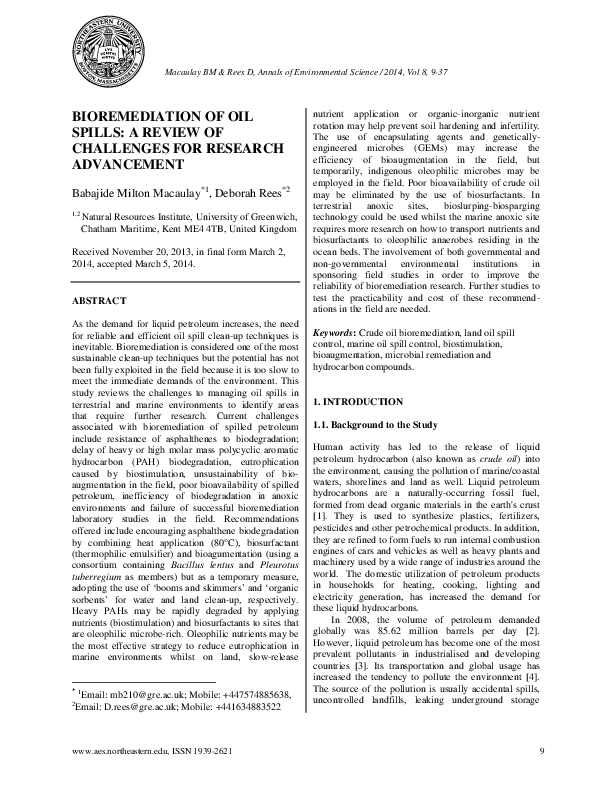
BIOREMEDIATION OF OIL SPILLS: A REVIEW OF CHALLENGES FOR RESEARCH ADVANCEMENT

2014, Annals of Environmental Science
As the demand for liquid petroleum increases, the need for reliable and efficient oil spill clean-up techniques is inevitable. Bioremediation is considered one of the most sustainable clean-up techniques but the potential has not been fully exploited in the field because it is too slow to meet the immediate demands of the environment. This study reviews the challenges to managing oil spills in terrestrial and marine environments to identify areas that require further research. Current challenges associated with bioremediation of spilled petroleum include resistance of asphalthenes to biodegradation; delay of heavy or high molar mass polycyclic aromatic hydrocarbon (PAH) biodegradation, eutrophication caused by biostimulation, unsustainability of bioaugmentation in the field, poor bioavailability of spilled petroleum, inefficiency of biodegradation in anoxic environments and failure of successful bioremediation laboratory studies in the field. Recommendations offered include encouraging asphalthene biodegradation by combining heat application (80°C), biosurfactant (thermophilic emulsifier) and bioagumentation (using a consortium containing Bacillus lentus and Pleurotus tuberregium as members) but as a temporary measure, adopting the use of ‘booms and skimmers’ and ‘organic sorbents’ for water and land clean-up, respectively. Heavy PAHs may be rapidly degraded by applying nutrients (biostimulation) and biosurfactants to sites that are oleophilic microbe-rich. Oleophilic nutrients may be the most effective strategy to reduce eutrophication in marine environments whilst on land, slow-release nutrient application or organic-inorganic nutrient rotation may help prevent soil hardening and infertility. The use of encapsulating agents and genetically-engineered microbes (GEMs) may increase the efficiency of bioaugmentation in the field, but temporarily, indigenous oleophilic microbes may be employed in the field. Poor bioavailability of crude oil may be eliminated by the use of biosurfactants. In terrestrial anoxic sites, bioslurping-biosparging technology could be used whilst the marine anoxic site requires more research on how to transport nutrients and biosurfactants to oleophilic anaerobes residing in the ocean beds. The involvement of both governmental and non-governmental environmental institutions in sponsoring field studies in order to improve the reliability of bioremediation research. Further studies to test the practicability and cost of these recommendations in the field are needed.
Related Papers
Babajide Macaulay
Petroleum-contamination of both terrestrial and marine environments have persisted as a result of the increasing demand on liquid petroleum globally which has led to the need to clean up spilled petroleum using eco-friendly methods. Of all the petroleum-cleaning techniques explored, the use of petroleum-degrading microbes has received most attention. The microbial remediation of spilled petroleum has been proved to be cost-effective, eco-friendly and sustainable. However, these microbes have been found to thrive under certain environmental/nutritional conditions which influence their behaviour towards spilled petroleum. This study aims to identify the factors responsible for the change in behaviour of oil-degrading microbes which might help facilitate better petroleum spill management. Some of these factors include: the physical nature of the spilled petroleum; chemical nature of the spilled petroleum; availability of nutrients; water temperature; concentration of oxygen; soil region/soil particle size; competition from other micro-organisms. Petroleum-degrading microbes were also found to degrade specific hydrocarbon components in liquid petroleum due to the specific metabolic pathway utilized by individual microbes. This makes the use of a microbial consortium a more aggressive option for the microbial degradation of spilled petroleum than the use of microbial isolates. However, more research on the factors influencing theabundance and productivity of oil-degrading anaerobes may need to be carried out. Also, how oil-degrading microbes can be aided to break down asphalthenes should be investigated.
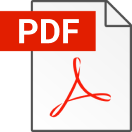
Biodegradation
Carla C C R de Carvalho , M Manuela da Fonseca , Meenu Tyagi
Journal of Chemical Technology & Biotechnology
Maria Nikolopoulou , Nicolas Kalogerakis
Mohamed Hussein
Stimulation of indigenous degraders with suitable nutrient s can significantly enhance bioremediation rates of marine environments polluted with petroleum hydrocarbons. Biostimu lation is emerging as the best strategy for combating oil spills following first response actions. This mini review is focused on t he conditions under which biostimulation leads to increased effectiveness and strategies for successful biostimulation to fresh and chronically polluted sites are suggested.
Coastal and Deep Ocean Pollution
Lautaro Girones , Analía Serra
Remediation of marine systems, which could be polluted by both organic and inorganic contaminants, is a complex process. Traditional remediation strategies include chemical, physico-chemical and thermal techniques, which are still widely used. However, biological techniques have begun to be applied, as they are eco-friendly and low cost alternatives. Biological remediation includes bioremediation and phytoremediation, which are defined as the use of microorganisms and plants, respectively, to remediate polluted sites. This chapter first describes briefly the physico-chemical factors that can affect bioremediation and phytoremediation and, in the case of bioremediation, the microorganisms that could be involved. Then, the different bioremediation (bioaugmentation, biostimulation, bioventing, bioleaching, etc.) and phytoremediation (phytoextraction, phytostabiliazation, phytovolatilization, etc.) strategies are defined. Finally, emphasis is placed on the biological remediation of marine systems, both in seawater and in sediments. This part is divided into inorganic (namely metals) and organic (oil spills and persistent organic pollutants) pollutants, discussing the different bioremediation and phytoremediation strategies that are applied or could be applied in marine systems. Combinations of techniques and novel approaches including genetic engineering are also considered.
Microbial Action on Hydrocarbons
Milene Gomes
Petroleum pollution is an environmental issue often reported, including oil spills that occur accidentally worldwide. The release of large quantities of oil causes directly or indirectly huge environmental and economic impacts and may persist for decades. Bioremediation processes, such as biostimulation and bioaugmentation, among others, represent an eco-friendly and effective way to treat impacted areas based on the use of biological agents, associated or not to other compounds like biosurfactants in order to mineralize or complex organic and inorganic pollutant compounds. Therefore, this book chapter will review some topics related to bioremediation, including several in situ and ex situ techniques employed to treat polluted areas and the use of biosurfactants produced by several microorganisms. Moreover, oil spills and how they can affect marine and terrestrial environments are also mentioned, based on recent reports available in literature and according to organizations responsible for environmental impact monitoring. Hydrocarbonoclastic microorganisms have been described in both environments as well as the community dynamics of specific groups as a function of oil compounds input. In marine environments, a high abundance increase of a specific group called “obligate hydrocarbonoclastic bacteria (OHCB)” has been reported after an event involving petroleum contamination. Similar observation has been reported for mangroves, showing that oil or its derivatives allow the selection of microorganisms capable to degrade hydrocarbons. Petroleum contamination in cold environments, as Arctic and Antarctic regions, represents a huge challenge since management of contaminated sites and bioremediation effectiveness in these regions depend on several factors influencing oil degradation under cold conditions facing intrinsic limiting factors. In conclusion, bioremediation is not only a scientific concept described in literature but a concrete and applied efficient tool to treat polluted environments. The increasing number of bioremediation companies and patents also corroborates the tendency in search for new technologies and approaches focusing on sustainable management of polluted areas.
Jacob Alberto Valdivieso Ojeda
Andrea M. Garcia
Marine Pollution Bulletin
European Journal of Soil Biology
RELATED PAPERS
The Environmentalist
Daniela Tovar
Editor CSRL
Yeti Darmayati
Dr. Abdullah M El Mahdi
Salvador Ordóñez
Colin Cunningham
Journal of Applied Microbiology
Jacqueline Stroud
Annie Monanga
Carlos Navarro Laguna
Lagos State University Journal
Olumide S Omotosho
José Gallegos
Journal of Hazardous Materials
Joaquim Vila
Extremophiles
Jackie Aislabie
IJAR Indexing
Chinedu Nwufo
Reviews of Environmental Contamination and Toxicology
Venkateswarlu Kadiyala , M. Megharaj
Suzan Pantaroto De Vasconcellos
Diane Wagenbrenner-Bush , patricia hilgard
International Biodeterioration & Biodegradation
Environment International
Mallavarapu Megharaj
Adeyemi Azeem
Panos GKOREZIS , Panagiotis Gkorezis
Environmental Science and Pollution Research
Rodolfo Marsch
Bioremediation Journal
Susana Vazquez
Barbara Wiseman , Diane Wagenbrenner-Bush , patricia hilgard
sciepub.com SciEP
Pure and Applied Chemistry
Randhir Makkar
mohamed abd el gawad
Environmental Microbiology
Frédéric Coulon
Saif N Al-Bahry
Polar Biology
SHEKHAR P TAMANI
IOSR Journals publish within 3 days
Maria Nikolopoulou
RELATED TOPICS
- We're Hiring!
- Help Center
- Find new research papers in:
- Health Sciences
- Earth Sciences
- Cognitive Science
- Mathematics
- Computer Science
- Academia ©2024

An official website of the United States government
The .gov means it’s official. Federal government websites often end in .gov or .mil. Before sharing sensitive information, make sure you’re on a federal government site.
The site is secure. The https:// ensures that you are connecting to the official website and that any information you provide is encrypted and transmitted securely.
- Publications
- Account settings
Preview improvements coming to the PMC website in October 2024. Learn More or Try it out now .
- Advanced Search
- Journal List

Recent Strategies for Bioremediation of Emerging Pollutants: A Review for a Green and Sustainable Environment
1 Department of Microbiology, Punjab Agriculture University, Ludhiana 141001, India
Diksha Garg
Banjagere veerabhadrappa thirumalesh.
2 Microbial Processes and Technology Division, CSIR-National Institute for Interdisciplinary Science and Technology, Thiruvananthapuram 695019, India
Minaxi Sharma
3 Laboratoire de Chimie Verte et Produits Biobasés, Département Agro Bioscience et Chimie, Haute Ecole Provinciale de Hainaut-Condorcet, 11 Rue de la Sucrerie, 7800 Ath, Belgium
Kandi Sridhar
4 UMR1253, Science et Technologie du Lait et de l’œuf, INRAE, L’Institut Agro Rennes-Angers, 65 Rue de Saint Brieuc, F-35042 Rennes, France
Baskaran Stephen Inbaraj
5 Department of Food Science, Fu Jen Catholic University, New Taipei City 24205, Taiwan
Manikant Tripathi
6 Biotechnology Program, Dr. Rammanohar Lohia Avadh University, Ayodhya 224001, India
Associated Data
Data that support these findings available within the article.
Environmental pollution brought on by xenobiotics and other related recalcitrant compounds have recently been identified as a major risk to both human health and the natural environment. Due to their toxicity and non-biodegradability, a wide range of pollutants, such as heavy metals, polychlorinated biphenyls, plastics, and various agrochemicals are present in the environment. Bioremediation is an effective cleaning technique for removing toxic waste from polluted environments that is gaining popularity. Various microorganisms, including aerobes and anaerobes, are used in bioremediation to treat contaminated sites. Microorganisms play a major role in bioremediation, given that it is a process in which hazardous wastes and pollutants are eliminated, degraded, detoxified, and immobilized. Pollutants are degraded and converted to less toxic forms, which is a primary goal of bioremediation. Ex situ or in situ bioremediation can be used, depending on a variety of factors, such as cost, pollutant types, and concentration. As a result, a suitable bioremediation method has been chosen. This review focuses on the most recent developments in bioremediation techniques, how microorganisms break down different pollutants, and what the future holds for bioremediation in order to reduce the amount of pollution in the world.
1. Introduction
Pollution of the environment, freshwater, and topsoil has evolved from global industrialization. Water quality has worsened as a result of human activity, such as due to mining and ultimate removal of toxic metal effluents from steel mills, battery companies, and electricity generation, posing major environmental concerns. Effluents like petroleum, polythenes, and trace metals harm the environment. Heavy metals are pollutants that exist in nature in the Earth’s crust and are difficult to decompose. They exist as ores in rocks and are recovered as minerals. High-level exposures can release heavy metals into the environment. Once in the environment, they remain toxic for much longer [ 1 ]. Many of these pollutants are mutagenic to both humans along with their surroundings. Absorbing heavy metals accumulates in the brain, liver, and kidney. Other effects on animals include cancer, nervous system damage, stunted growth, and even death [ 2 ]. Heavy metals in soils reduce food quality and quantity by inhibiting nutrient absorption, plant growth, and physiological metabolic processes. Metal-contaminated soils are being remedied using chemical, biological, and physical methods. However, physicochemical methods produce a lot of waste and pollution, so they are not valued [ 3 ]. Bioremediation is a cost-effective and practical solution for removing environmental contaminants [ 4 ]. Plant growth promotion, insect control, soil conservation, nutrient recycling, and pollutant reduction are all key functions of soil microorganisms [ 5 ]. Bioremediation has come a long way in terms of efficiency, cost, and social acceptability [ 6 ]. Bioremediation research has largely focused on bacterial processes, which have numerous applications. Archaea are known to play a role in bioremediation in many applications where bacteria are involved. Many hostile situations have degraded, requiring bioremediation. Microbes can also assist in the elimination of pollutants from hyperthermal, acidic, hypersaline, or basic industrial waste [ 7 , 8 ]. Recent research suggests that using more than one living organism will improve the efficiency and results, and allow for greater microbial diversity in bioremediation [ 8 , 9 ]. Many researchers employed bioremediation technology for the removal of organic and inorganic pollutants [ 10 , 11 , 12 ]. In a study, bioremediation technology was used for the treatment of various pollutants, including organophosphate pesticides such as chlorpyrifos, methyl parathion, and profenofos, by Aspergillus sydowii , and chloramphenicol by endophytic fungi, respectively [ 13 , 14 ]. In another study, Cymbella sp. has been shown to detoxify naproxen-polluted water with an efficiency of 97.1% [ 15 ].
A bioremediation approach requires the use of microbial enzymes to break down hydrocarbons into less harmful compounds. The widespread use of genetically-modified microorganisms that can also help to eliminate petroleum, naphthalene, toluene, benzene, and other xenobiotic chemicals is now being studied [ 16 ]. Several factors, such as temperature of the surrounding environment, aerobic or anaerobic conditions, and nutrient availability, all influence bioremediation for better outcomes. Emerging environmental pollutants, such as persistent organic compounds, heavy metals, toxins, and air pollutants that are of synthetic or natural origin, reach ecosystems mainly through anthropogenic activities and pose adverse threats to lifeforms like plants, animals, and humans [ 17 ]. One of the most economical and environmentally favorable biotechnological innovations is bioremediation. Waste management mainly relies on bioremediation. It can remove persistent organic pollutants, which are hard to breakdown and are thought to be heterologous biological substances. This review addresses the recent approaches and updated information of bioremediation strategies for eco-friendly detoxification and the effective degradation of various organic and inorganic contaminants to control environmental pollution.
2. Microorganisms Used in Bioremediation
Biological equilibrium is maintained in part by the contribution of microorganisms to nutritional chains. Bioremediation is the process of using bacteria, algae, fungi, and yeast to remove contaminated materials from the environment [ 18 ]. In the presence of hazardous compounds or any waste stream, microbes can grow at temperatures as low as −196 degrees Fahrenheit and as high as 1200 degrees Fahrenheit. The adaptability and biological systems of microbes make them an ideal choice for remediation [ 19 ]. Carbon is the most important nutrient for microorganisms. Microbes from a variety of environments were used to perform bioremediation. Achromobacter , Alcaligenes , Xanthobacter , Arthrobacter , Pseudomonas , Bacillus , Mycobacterium , Corynebacterium , Flavobacterium , Nitrosomonas , and other microorganisms [ 9 ] are examples of microbes.
2.1. Aerobic
Several microorganisms have the ability to bioremediate different types of environmental pollutants under aerobic conditions. Bacillus , Pseudomonas , Sphingomonas , Flavobacterium , Nocardia , Rhodococcus , and Mycobacterium are aerobic bacteria that can degrade a variety of complex organic compounds [ 20 ]. Pesticides, alkane hydrocarbons, and polyaromatic compounds have been shown to be degraded by these microbes. Several of these microorganisms make use of these contaminants as a source of carbon and energy [ 21 ]. In the aerobic bioremediation process, oxygen is the limiting factor for the growth of microorganisms.
2.2. Anaerobic
Amphibious bacteria that degrade and convert pollutants to fewer toxic forms are becoming increasingly popular for the bioremediation of polychlorinated biphenyls, chlorine compounds, and the chlorinated solvents, trichlorethylene and chloroform [ 22 ]. Several bacteria, such as Pseudomonas , Aeromonas, and sulfate-reducing bacteria, have been used in the bioremediation process under anaerobic conditions. Garg and Tripathi [ 23 ] reported microbial discoloration of azo dyes under different environmental situations. Azo dyes can decompose anaerobically through reduction reactions using electrons produced by the oxidation of the organic substrate(s). Due to such controlled dye decolorization events, microbe electrochemical properties would have a major impact on the effectiveness of color removal. Dyes were anaerobically decolored for industrial activities to progressively acquire such time-variant decolorized-metabolites (DMs). However, external augmentation of DMs gathered under certain conditions was carried out for improved research so that a precise system can be used [ 24 ].
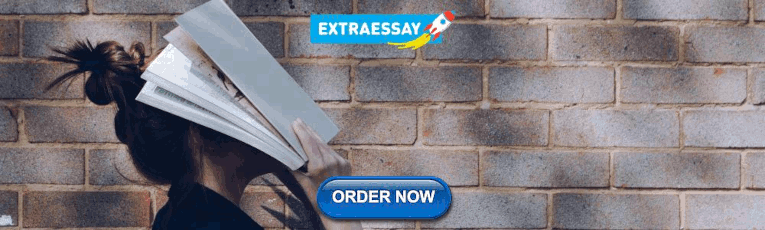
3. Factors Affecting Microbial Bioremediation
Bioremediation is the process of using microorganisms such as bacteria, algae, fungi, and plants to break down, change, remove, immobilize, or detoxify various physical and chemical pollutants in the environment. Microorganisms’ enzymatic metabolic pathways speed up biochemical reactions that break down pollutants [ 25 , 26 ]. In order for microorganisms to combat pollutants, they must come into contact with compounds that provide them with the energy and nutrients they need to multiply. There are several factors such as physical, chemical, biological, soil-type, carbon and nitrogen source, type of microorganisms—i.e., single or consortium—and others that affect the process of bioremediation [ 27 ]. Microbial consortiums often have both multifunctionality and resistance because different species work together to use all substrates in the best way possible, thereby increasing the bioremediation efficiency compared to single microorganism [ 28 ]. In a study, carbon is one of the most important nutrients that help in situ bioremediation by increasing the metabolic activity of natural microbial communities and speeding up the bioremediation process to break down existing pollutants. Bioremediation may use organic carbon more than any other additive. In an anaerobic environment, many microorganisms can ferment organic carbon and make hydrogen gas [ 29 ]. In a study, bioremediation was found to be significantly affected by soil types, and the removal efficiency of pollutants varied in sandy soil and clay soil, respectively [ 30 ]. For bioremediation to be a success, it must be able to access existing microorganisms as well as the environment’s physicochemical characteristics ( Table 1 ). The microbial population responsible for degrading pollutants, the accessibility of contaminants, and the following factors are taken into consideration.
Critical factors for microbial bioremediation.
4. Principle of Bioremediation
When organic wastes are biologically degraded under controlled conditions, “bioremediation” is the term used to describe this process. Using bioremediation, harmful substances can be degraded or detoxified by providing the organisms with the nutrients and other chemicals they need to function optimally. Enzymes play a critical role in every stage of the metabolic process [ 24 , 43 ]. It is part of the family of oxidoreductases, lyases, transferases, and hydrolases. Non-specific and specific substrate affinities allow many enzymes to degrade a wide range of substrates. There must be enzymatic action on the pollutants in order for bioremediation to be successful. In order to speed up microbial growth and degradation, environmental parameters must often be manipulated during bioremediation [ 38 , 43 ]. This is because bioremediation only works when the environment is right for microbes to grow and move around.
Living organisms and fertilizers can aid in the process of bioremediation, which occurs naturally and is encouraged. Biodegradation is a key component of bioremediation technology. It’s the process of converting harmful organic pollutants like carbon dioxide and water into non-toxic or naturally-occurring inorganic compounds that are safe for use by humans, plants, animals, and aquatic life [ 44 ].
5. Types of Bioremediations
Bioremediation can be used in a plethora of ways, and some of the most commonly used methods are presented here ( Figure 1 ).

Diverse bioremediation techniques.
5.1. Biopile
In bioremediation, aeration and nutrient supplementation are used to enhance microbial metabolic activities in the piled-up polluted soil above ground. Aeration, nutrients, irrigation, leachate collection, and treatment bed systems are all included in this procedure. When it comes to ex situ biodegradation, this method is becoming increasingly popular because of its cost-effectiveness and useful features, such as pH and nutrient control. Using the biopile to clean up polluted cold environments and treat low-molecular-weight volatile pollutants is an option [ 15 , 45 ]. The biopile’s adaptability allows for a reduction in remediation time by increasing microbial activity and contaminant availability while also increasing biodegradation rate. When warm air is introduced into the biopile system to provide air and heat simultaneously, bioremediation is improved. The biopile’s remediation process has been helped by the addition of bulking agents like straw, sawdust, or wood chips. To replenish the air supply to polluted piled soil in biopiles, ex situ bioremediation techniques such as land farming, biosparging, and bioventing can be applied [ 46 ]. However, these techniques are expensive to implement and require a power supply at remote locations. Bioremediation may be slowed down by extreme air temperatures that dry soil and make it more likely to be vaporized than to be broken down by living organisms [ 47 ]. Bio-available organic carbon (BOC) plays an important role in bioremediation through the biopile method. Petroleum contaminated soil has been bioremediated using mesophillic conditions (30 °C–40 °C) and a low aeration rate for the removal of total petroleum hydrocarbon (TPH) using alpha , beta , and gamma proteobacteria [ 48 ]. Biopile systems have also been utilized for treating the diesel contaminated soil of the sub-Antarctic region. A total of 93% of the total petroleum hydrocarbon (TPH) was removed using the biopile system within one year [ 49 ].
5.2. Windrows
Windrows boosts bioremediation by enhancing the biodegradation processes of native and transitory hydrocarbon plastic found in the contaminated soils when spinning the heaped contaminated soils. The aeration, mineralization, and biotransformation of toxic soil can be performed through acclimation, biological treatment, and mineralization [ 50 ], can speed up bioremediation. The biopile approach can remove more hydrocarbons from soil than windrow treatment [ 15 , 51 ], which was more efficient in terms of hydrocarbon removal. The periodic rotation connected with windrow remediation is not a better selection approach for the bioremediation of soil affected by harmful volatile chemicals. Windrow treatment is a source of greenhouse gas (CH 4 ) due to the anaerobic system generated inside the heaped contaminated soil [ 52 ]. The windrow method of has been applied for the bioremediation of the Gurugram–Faridabad dumpsite in Bandhwari, India by forming terraces and windrows and utilizing bio-culture, and the results showed a decrease in the garbage [ 53 ].
5.3. Land Farming
Land farming is the most significant and simple bioremediation method because of its low operating costs and lack of specialized equipment [ 54 ]. Ex situ bioremediation is the most common method, but it can also occur with in situ bioremediation. The reason for this is the location of the treatment. It is common practice in land farming to remove and till polluted soils on a regular basis, and the location of treatment dictates the type of bioremediation. On-site treatment is classified as in situ , whereas ex situ bioremediation approaches are used for the treatment of the contaminated soil [ 55 ]. Extracted contaminated soils are usually placed on a permanent layer of substrate well above Earth’s surface to permit native microorganisms to aerobically degrade contaminants [ 56 ]. Land bioremediation of polluted soil using land farming bioremediation technology is a reasonably simple process that takes little capital, has little ecological footprint, and uses very little energy [ 57 ].
5.4. Bioreactor
Following a series of biological reactions, bioreactors transform raw materials into specific products. Bioremediation thrives in a bioreactor, which provides the ideal conditions for growth [ 58 ]. The remediation samples are placed in a bioreactor. There are a number of advantages to using a bioreactor to treat contaminated soil as opposed to ex situ bioremediation methods. An efficient bioremediation process based on bioreactors that can precisely regulate pH, agitation, temperature, aeration, substrate concentration, and inoculum concentration significantly reduce the time required for bioremediation [ 59 ]. Biological reactions can take place when the bioreactor can be controlled and manipulated. Given their adaptability, bioreactor designs are able to maximize microbial degradation while abiotic losses are kept to a minimum.
In Situ Bioremediation Techniques
These methods entail cleaning up polluted substances right where they were created. It does not necessitate any digging or disturbance of the surrounding soil. These techniques ought to be more cost-effective in comparison to the ex situ bioremediation techniques. Bioventing, phytoremediation, and biosparging are examples of in situ bioremediation techniques that can be improved, while intrinsic bioremediation and natural attenuation are examples of in situ bioremediation techniques that cannot be improved [ 60 ]. In situ bioremediation approaches have effectively treated chlorine, paints, toxic metals, and hydrocarbon-contaminated areas [ 61 ]. The practice of in situ bioremediation can be categorized into two distinct types: intrinsic and engineered.
- (a) Intrinsic in situ bioremediation:
Natural reduction is another term for in situ bioremediation. Intrinsic bioremediation utilizes polluted sites in a non-invasive manner (human intervention) [ 62 ]. The goal of this procedure is to stimulate an already existing microbial population. The biodegradation of polluting constituents, including those that are recalcitrant, is based on aerobic and anaerobic processes in microorganisms. It costs less because there isn’t a lot of force behind this technique [ 63 ]. Intrinsic in situ bioremediation can be performed using anaerobic reductive dechlorination, aerobic treatment, amendment delivery, biosparging, and bioslurping [ 64 ]. Using a stimulation–optimization approach that is powered by machine learning and particle swarm optimization (ELM–PSO) techniques, in situ bioremediation has been used as a method for the biological treatment of clogged groundwater [ 65 ]. This technique was implemented through the use of in situ bioremediation. This results in cheaper technology for the pumping system and requires less capital for the whole process. The concentration of contaminants was reduced from 40 ppm to 5 ppm (within permissible range) in 3 years using in situ bioremediation. In situ remediation has also been explored for the decontamination of Cr (VI) found in shallow unsaturated soil. Microorganisms possess the capability to survive under high concentrations of Cr (VI) in the soil and their sub-cellular machinery was utilized to interact with heavy metals. Microbial inoculants can be utilized for the in situ treatment of heavy metals [ 66 ]. Cr (VI) interacts with Fe (II) ions also through the redox reactions, and the release of iron in soluble forms promotes the reductive reactions [ 67 ].
- (b) Engineered in-situ bioremediation
In the second method, a specific microorganism is brought into the area of contamination to clean it up. In situ bioremediation is a technique that employs microorganisms that have undergone genetic engineering in order to hasten the decomposition process. This is accomplished by enhancing the physicochemical conditions that foster the growth of microorganisms [ 68 ].
5.5. Bioventing
Bioventing is a technique that uses controlled airflow to increase the activity of indigenous microbes for bioremediation by delivering oxygen to the unsaturated zone. The bioremediation process is aided by the addition of nutrients and moisture during the bioventing process. This will lead to the microbial transformation of pollutants into harmless substances. Other in situ bioremediation methods have flocked to this one in recent years [ 69 ]. Bioventing is a technique that helps in stimulating the indigenous microflora through ample amounts of aeration to enhance the biodegradation ability of the various microbes and promote decontamination of the heavy metal pollutants by precipitation [ 70 ].
5.6. Bioslurping
A direct oxygen supply and stimulation of contaminant biodegradation are used in conjunction with vacuum-assisted pumping, bioventing, and soil vapour extraction (SVE) in order to reach soil and groundwater levels for restoration [ 71 ]. This approach can be used to recover unsaturated and saturated zones as well as light non-aqueous phase liquids (LNAPLs). This technology can be used to remediate soils contaminated with flammable and moderately-flammable organic substances. Liquid is drawn from the free product layer by means of a “slurp” that spreads into the layer. LNAPLs are lifted to the surface by the pumping machine, where they are separated from the surrounding air and water [ 72 ]. To reduce microbial activity, soil moisture is used in this technique to reduce air permeability and oxygen transfer rate. Given that it uses less groundwater, this method saves money on storage, disposal, and treatment, even though it’s not ideal for remediation in low-permeable soils. Bioslurping requires 25 feet of digging below the ground surface and then the contaminants floating on the water can be removed. It combines both the approaches of bioventing, which utilize aerobic bioremediation of contaminated soil in situ. Free product is recovered by a vacuum-enhanced system that utilizes LNAPLs from the capillary fringe [ 73 ]. Free product is “slurped” up the bioslurping tube into a trap or oil–water separator for further treatment after the bioslurping tube is vacuumed. When the LNAPL is removed, the height of the LNAPL drops, which encourages the flow of LNAPL from distant locations into the bioslurping well. The bioslurping tube starts to remove vapours from the unsaturated zone when the fluid level in the bioslurping well decreases as a result of the vacuum extraction of LNAPL. This vapour extraction encourages soil gas movement, which in turn boosts aerobic biodegradation and aeration [ 74 ].
5.7. Biosparging
Air is introduced into the soil’s core, just like bioventing, to encourage microbiological activity, which in turn removes pollutants from polluted sites. As an alternative to conventional biodegradation methods, bioventing involves injecting air into a saturated zone in order to encourage the movement of flammable organic chemicals upward to an unsaturated zone nearby [ 75 ]. The success of biosparging is dependent on soil porosity and contaminant biodegradability. When it comes to bioventing and soil vapour extraction (SVE), in situ air sparging (IAS) uses high air-flow rates to volatilize contaminants, while biosparging encourages microbial degradation [ 76 ]. It is common practice to use biosparging to remove diesel and kerosene from water supplies. In order to hasten the biodegradation processes, oxygen is supplied into microorganisms during enhanced bioremediation [ 77 ]. The removal of organic pollutants (BTEX) can be accomplished using a variety of technologies, including adsorption, microbial degradation, biosparging, PRBs, and the use of modified or synthesized zeolites. However, there aren’t many investigations on readily available, inexpensive materials like natural zeolite for BTEX adsorption [ 78 ].
5.8. Phytoremediation
Contaminated soils can be cleaned up using phytoremediation. In contaminated areas, this method uses plant interactions at the physical, biological, chemical, biochemical, and microbiological levels to reduce pollutant toxicity. Depending on the quantity and form of the pollutant, phytoremediation employs a variety of processes [ 79 ]. Extraction, sequestration, and transformation are common methods for removing pollutants like heavy metals. When using plants like willow or alfalfa, the decay, immobilization, rhizoremediation, and evaporation of organic contaminants such as oils and chloro-compounds is feasible [ 80 ]. Tap root system or fibrous root system, penetration, toxicity levels, adaptability to the harsh environmental conditions of the contaminants, plant annual growth, supervision, and, notably, the time needed to reach standard of cleanliness are all important factors in plants that serve as phytoremediators. The plant must also be disease and insect resistant [ 81 ]. An important part of phytoremediation is removing pollutants from the roots and shoots. The movement of water and nutrients is also dependent on transpiration and partitioning [ 82 ]. When it comes to contaminants and plant nature, it is possible to alter this process. Phytoremediation can be accomplished with the help of the majority of the plants present at a polluted site. In polluted environments, native plants can be bioaugmented by natural or anthropogenic plants, or a combination of both. Phytomining, the process of extracting precious metals from polluted sites with plants, is one of them [ 83 ].
Numerous plants (over 300) are better candidates for phytoremediation because they ideally absorb Cu, Zn, and Ni. Phytostabilization, sometimes referred to as in situ inactivation or immobilisation of heavy metals, reduces their bioavailability and prevents their off-site transfer. At the plant roots, it absorbs metals and restores them. Several species, notably Acanthus ilicifolius and Virola surinamensis , are capable of Cd photostability. Cinnamomum camphora , Osmanthus fragrans , Euonymus japonicus , Ligustrum vicaryi , and Loropetalum chinense are five decorative plants chosen for their capacity to phytostabilize Cd [ 84 ]. Water from various places that has been contaminated with metal can be successfully treated using bacterially-aided phytoremediation. The phytoremediation method of metal reduction in wastewater utilising plants can be used by coalitions of growth-promoting rhizobacteria, degrading bacteria, as well as endophytic bacteria [ 85 ]. There are a few limitations to bioremediation techniques, as presented in Table 2 .
Limitations of various bioremediation techniques.
6. Bioremediation of Various Pollutants
6.1. bioremediation for organic pollutants.
Organic compounds (OCs) such as biocides and flame retardants have been widely used and are now considered a threat to nearly all forms of life on the planet because of the widespread and massive use of these chemicals in the environment. Most OCs, such as polychlorinated biphenyls (PCBs), polybrominated biphenyl ethers (PBEs), and polycyclic aromatic hydrocarbons (PAHs), can be degraded in the environment by microbes. Biodegradation is the process by which microbes break down organic compounds into less toxic or entirely non-toxic residues [ 91 ]. In order to obtain organic carbons and energy, the microbes consume the organic substrate. Isolated from other microbes, an individual microbial species usually does not degrade any organic substrate [ 92 ] and does well in a community. As a result of community microbe interactions, resistance, chemical-degrading ability, and tolerance are all conferred by the exchange of genetic information among microbial species. Many OC-degrading microorganisms are misidentified due to a lack of internationally agreed-upon methods and protocols for microbial identification [ 93 ]. This underlines the significance of studies into microbial consortiums using metagenomics tools and conventional genetic engineering protocols. Bacteria and other microorganisms have the ability to degrade a wide range of organic compounds, depending on the chromosomal genes, as well as the extracellular enzymatic activity (in the case of bacteria) (fungal degradation process). The varying environmental conditions that affect the microbe growth pattern further complicate these processes [ 94 ].
A successfully bioengineered microbe requires the identification of the relevant species and strains for each substrate. A viable alternative to the recombinant degradation of resistant organic compounds is biodegradation by microbes using readily-available organic carbon and energy sources in the surrounding environment. Microbes use the fluctuation in chemical gradients in their environment to determine the most favourable conditions for growth. This allows them to thrive in an optimal environment [ 95 ]. Microbial consortia and microbial fuel cells (MFCs and bioreactors) are two new developments in microbiological bioremediation that are being used to degrade recalcitrant organic compounds. Toxic organics can be remedied more effectively using fungi rather than bacteria because the latter cannot grow at high concentrations of toxic organics [ 96 ]. For example, the enzymes, laccase (LAC), lignin peroxidase (Lip), and manganese decarboxylase (MDA), are active in the metabolism of lignocellulosic compounds by the white rot fungus Phanerochaete chrysosporium [ 97 ].
6.2. Bioremediation for Inorganic Pollutants
Toxic heavy metals and their compounds resulting from mining, power plants, metallurgy, and chemical manufacturing processes are among the most common inorganic contaminants [ 98 ]. One of the main concerns of environmentalists is toxic elemental pollution because the disposal of toxic metals to soils and waters on or below the surface causes unacceptable health risks [ 99 ]. Microbes cannot degrade metal ions; it is essential to know that they are only capable of changing the oxidation states of the metals to stabilize them [ 100 ]. They can metabolize and detoxify metals like any other nutrient in the cells. Several microorganisms have been reported for the bioremediation of organic and inorganic pollutants ( Table 3 ). Microbes that release chelating agents and acids, as well as those that alter physicochemical properties such as redox potential in their environment can cause significant changes in the environment by increasing the bioavailability of metal ions [ 101 ]. Physical adsorption, biosorption, and ion complexation are the first steps in the interaction between metals and microbial cells [ 102 ]. Enzymes for oxidation, methylation, reduction, precipitation, and dealkylation are involved in the biochemical transformation of metal ions by microorganisms. The adaptability of microbes to heavy metals, such as iron, zinc, chrome, magnesium, mercury, and barium in textile waste, was demonstrated in the multidrug-resistant Pseudomonas aeruginosa T-3 isolate from tannery effluent [ 67 , 83 ]. This shows that microbes can adapt to changing environmental conditions. A plasmid-encoded copper and cadmium metal resistance gene in the bacteria, Pseudomonas putida PhCN, has also been discovered [ 103 ]. Plasmid-encoded biochemical information and genetic engineering techniques were used to create recombinant Escherichia coli that expresses the metallothionein gene ( Neurospora crasa ) for Cd uptake, resulting in significantly faster Cd uptake than the donor microbe [ 104 ]. A poly-histidyl peptide was introduced into Staphylococcus xylosus and Staphylococcus carnosus that encoded genes that allowed these microbes to bind nickel [ 105 ].
Potentially hazardous organic and inorganic pollutants and their degrading microbes (bacteria, fungi, and algae).
7. Recent Advancement and Challenges in Bioremediation
7.1. bioinformatics approaches in bioremediation.
When it comes to waste management, bioremediation is a useful technique that can be used to remove waste from contaminated areas and sites. It is particularly concerned with the utilization of organisms to consume or neutralize pollutants [ 20 ]. Using data from various biological databases, such as databases of chemical structure and composition, RNA/protein expression, organic compounds, catalytic enzymes, microbial degradation pathways, and comparative genomics to interpret the underlying degradation mechanism carried out by a particular organism for a specific pollutant is the goal of bioremediation [ 133 ]. A variety of bioinformatics tools are used to interpret all of these sources in order to study bioremediation in order to develop more effective environmental cleaning technology. There has been a scarcity of data on the factors that control the growth and metabolism of microbes with bioremediation potential, which has resulted in a limited number of bioremediation applications [ 134 ]. These microorganisms with bioremediation capabilities have been profiled and their mineralization pathways and mechanisms have been mapped out using bioinformatics [ 135 ]. The use of proteomic approaches such as two-dimensional polyacrylamide gel electrophoresis, microarrays, and mass spectrometry is also critical in the investigation of bioremediation methods and technologies. It significantly improves the structural characterization of microbial proteins that have contaminant-degradable properties, according to the researchers [ 135 ]. The structural characterization of microbial proteins capable of degrading contaminants has greatly improved. Research in this field crosses the boundaries between computer science and biology. For example, computers are used to store, manipulate, and retrieve information linked to the DNA, RNA, and proteins of the genome [ 133 , 135 ].
7.1.1. Bioremediation Tools Based on Omics
Bioremediation studies can benefit from the use of genomics, transcriptomics, metabolomics, and proteomics. Given its ability to correlate DNA sequences with the abundance of metabolites, proteins, and mRNA, this technology aids in the in situ bioremediation process’s evaluation [ 136 , 137 ].
7.1.2. Genomics
There is a new field in genomics for the study of bioremediation microbes. This strategy is based on microbes’ ability to fully analyze their genetic information within the cell. Bioremediation uses a wide variety of microorganisms [ 138 ]. To better understand the biodegradation process, genomic tools such as PCR, analysis of isotope distribution, DNA hybridization, molecular connectivity, metabolic footprinting, and metabolic engineering are used. For genotypic fingerprinting, a variety of PCR-based techniques are available, including amplified fragment length polymorphisms (AFLP), amplified ribosomal DNA restriction analysis (ARDRA), automated ribosomal intergenic spacer analysis (ARISA), terminal-restriction fragment length polymorphism (T-RFLP), randomly amplified polymorphic DNA analysis (RAPD), single strand conformation polymorphism (SSCP), and length heterogeneity [ 139 ]. When it comes to studying soil microbial communities, RAPD can be utilized for assessing inherently related bacterial species, constructing functional structural models, and generating genetic fingerprints [ 140 ]. In microbial communities, LH-PCR may be used to detect natural length variations of various SSU rRNA genes. Multiple taxonomic groups of microbes can be profiled simultaneously using T-RFLP [ 141 ]. Research into how soil microbes interact with natural factors can also make use of a combination of molecular tools, such as genetic fingerprinting, microradiography, FISH, stable isotope probing, and quantitative PCR. A PCR-based quantitative analysis of soil microbial communities can be used to determine the abundance and appearance of taxonomic and operational gene markers in the soil. Techniques for analysing a person’s DNA use amplified PCR products as a starting point for the direct analysis of specific molecular biomarker genes [ 142 ]. In order to better understand the relationship between diverse microbial communities, cluster-assisted analysis, which compares fingerprints from different samples, could be used.
7.1.3. Transcriptomics and Metatranscriptomics
The transcriptome is a crucial link between cellular phenotype, interactome, genome, and proteome because it represents the set of genes that are being transcribed at a specific time and condition. The ability to control gene expression is critical to adapting to changes in the environment and thus ensuring survival. Transcriptomics provides a comprehensive view of this process across the entire human genome. In transcriptomics, DNA microarray analysis is a powerful tool for determining mRNA expression levels [ 143 ]. To perform a transcriptomic analysis, one must first isolate and enrich the total mRNA, then synthesize cDNA, and then sequence the cDNA transcriptome. Using a DNA microarray as a transcriptomics tool, almost every gene in an organism’s mRNA expression can be examined and studied [ 144 ]. The study of transcriptional mRNA profiles, also known as transcriptomics or metatranscriptomics, is critical for gaining functional insights into the activities of environmental microbial communities [ 145 ]. Syntrophism between microbes and complementary metabolic pathways can be discovered using metagenomics and genome binning as well as metatranscriptomics during the entire biodegradation process [ 146 ]. Metatranscriptomics is a way to look at gene expression that can be used by researchers [ 147 ].
7.1.4. Proteomics and Metabolomics
In contrast to metabolomics, which focuses on the total metabolites produced by an organism in a given period of time or environment, proteomics focuses on the total proteins expressed in a cell at a given location and time [ 148 ]. The analysis of protein abundance and changes in composition, as well as the identification of key microbe-related proteins, has been accomplished using proteomics [ 149 ]. In comparison to genomics, the functional analysis of microbial communities is more useful and holds more promise. There are two primary ways in which metabolomics studies can be used to analyze biological systems. It is not necessary to have any prior knowledge of the metabolic pathways of the biological system in order to conduct the first type of study. By employing this strategy, there are numerous metabolites in the sample that can be identified and recovered, which generates enormous amounts of data that can be used to establish the interconnectedness of various samples in metabolic pathways. Another option is to conduct a targeted study to identify specific metabolic pathways or metabolites based on prior research [ 150 ]. Metabolite profiling, foot printing, and target analysis are just some of the many tools in the toolbox of microbial metabolomics that can be used to identify and quantify the myriad of cellular byproducts present in living organisms [ 151 ]. Data from both the proteome and metabolome will be useful for cell-free bioremediation.
7.2. Bioremediation Using Nanotechnological Methods
A nanometer is the smallest unit of measurement used in nanotechnology. Many toxic substances can be removed with their help because of their unique abilities against various recalcitrant contaminants. Technology such as water treatment has been given a new perspective by nanotechnology. Techniques that are good for the environment can now be categorized as nanofiltration [ 151 ].
7.2.1. Microbe and Nanotechnology
When using effective microbes (EM) technology, wastewater can be treated with effective microbes, and the water can then be used for irrigation [ 152 ]. For water purification, nanotechnology and EM technology can be helpful. Innumerable and all-pervasive environmental issues arise from the presence of recalcitrant organic pollutants like polycyclic aromatic hydrocarbons (PAHs) with multiple benzene rings. Polycyclic aromatic hydrocarbons (PAHs) are mutagenic and non-biodegradable [ 153 ]. In a study, Ramos et al. [ 154 ] synthesized silver nanoparticles using whole cells of the fungi Trichoderma spp. for its application.
7.2.2. Engineered Polymeric Nanoparticles for Hydrophobic Contaminant Bioremediation
Soil sorption of organic pollutants, such as petroleum hydrocarbons and PAHs, reduces their solubility and mobility, which in turn reduces their environmental impact. The phenanthrene solubility and phenanthrene release from contaminated aquifer material are both improved by polymeric nano-network particles [ 155 ]. Precursor chains of poly-(ethylene) glycol-modified urethane acrylate (PMUA) are used to create polymeric nanoparticles. PMUA nanoparticles are designed to maintain their properties in the presence of a diverse range of bacterial populations [ 156 ].
7.3. Genetic and Metabolic Engineering
“Gene editing” refers to scientific technical developments that enable rational genetically-created fragments at genome level to provide exact addition, deletion, or substitution of pieces of DNA molecules. Transcription activators are utilized in a variety of gene editing methods, including TALENs, ZFNs, and CRISPRs, which are widely used in research. CRISPR-Cas has been dubbed the most efficient and straightforward gene editing tool [ 157 ]. A DNA-binding element in TALEN is complementary to the sequence of the host DNA. When TALEN attaches to DNA and exposes sticky ends for stabilization, it creates double-stranded breaks (DSBs). ZFNs also have a DNA-binding domain made up of 30 amino acids. At the target location of the host DNA, the Fok1 cleavage domain causes DSBs. A novel perspective on composite endonuclease comprising TALENs and ZFN nucleases was required to solve molecular problems [ 158 , 159 ]. Two of the CRISPR-Cas system’s unique properties are sequence similarity complementarity and simultaneous gene editing [ 160 , 161 ]. The bacteria, Streptococcus pyogenes, provides this unique ability as a sort of virus resistance. In the CRISPR-Cas system, guide RNA connects crisper-derived RNA (crRNA) and trans-acting antisense RNA (trcRNA). The Cas9 enzyme is able to carry out the requisite DSB when gRNA recognizes the target DNA sequence. These gene editing tools’ knock-in and knock-out effects are being analyzed for usage in bioremediation investigations [ 161 ]. In model organisms like Pseudomonas and Escherichia coli , the CRISPR-Cas system has been widely accepted by researchers [ 138 ]. In non-model species (such as Rhodococcus ruber TH, Achromobacter sp. HZ01, and Comamonas testosteroni ), the area of bioremediation is also exploring new insights into CRISPR toolkits and the synthesis of gRNA for the production of remediation-specific genes [ 162 ].
Pollutant-tolerant bacteria are the greatest choices for genetic manipulation and biochemical pathways since they are accustomed to tolerating and storing a variety of toxic, refractory, and non-degradable xenobiotic compounds under harsh circumstances. Furthermore, recognizing biochemical functions is critical for analyzing microbiological bioremediation, such as the bioremediation of harmful pollutants through the production of haloalkane dehalogenases and the disposal of pyrethrins from land through the anaerobic decomposition pathway of fenpropathrin studied in Bacillus sp. DG-02 [ 163 ]. The bioremediation process can be improved by metabolic engineering, which alters the existing pathway. The likelihood of obtaining recombinant enzymes increases significantly when using a genetic approach. Some extracellular enzymes have been found to play a role in enzymatic bioremediation, according to some studies. PAHs are degraded by LiPs ((lignin peroxidase) from P. chrysosporium that encode hemoproteins [ 164 ]. Even though contaminants can be consumed by microbes as substrates or intermediates in biological pathways, incomplete or partial degradation leads to simpler, non-toxic degradable compounds [ 136 ]. For example, LiP can degrade benzopyrene into three quinine compounds, namely 1,6-quinol, 3,6-quinine, and 6,12-quinine [ 165 ]. MnP (Mn (II) peroxidase) can also oxidise organic compounds in the presence of MnP (Manganese peroxidase) [ 166 ]. As well, laccase, glutathione S transferase, and cytochrome P 450 are involved in the biodegradation of recalcitrant compounds [ 167 ]. It has been shown that the immobilization of enzymes significantly increases enzyme stability, activity, and stability. Enzymatic bioremediation is a simple, environmentally-friendly, and fast method for removing and degrading persistent xenobiotic compounds by microorganisms [ 134 , 145 ]. Enzyme-producing microorganisms have been isolated and characterized with the limitation of low productivity. Insecticides’ main ingredients, organophosphates (OP) and organochlorines (OC), are found in agricultural soil and run-off into waterways.
Genetically-engineered microorganisms have demonstrated successful bioremediation of hexachlorocyclohexane and methyl parathion [ 135 , 146 ]. Genetically-modified P. putida KT2440 was used in organophosphate and pyrethroid bioremediation experiments [ 168 ]. The degradation and catabolism of a variety of persistent compounds has been documented since the advent of metabolic engineering. Sphingobium japonicum and Pseudomonas sp. WBC-3 showed bioremediation of methyl parathion and -hexachlorocyclohexane degradation pathways [ 169 ]. When three enzymes from two different microorganisms are combined in E. coli , a persistent fumigant called 1-, 2-, 3-trichloropropane is released into the environment via heterologous catabolism [ 137 , 148 ]. To do this, microbes can be used to turn persistent compounds into minerals [ 49 ].
7.4. Designing the Synthetic Microbial Communities
Synthetic biology advancements have had a significant impact on environmental issues in recent years. Toxic compounds, pesticides, and xenobiotics can be removed from the environment by using genetically-modified organisms (GMOs). Natural microbial communities must be understood in order to create a synthetic one [ 170 ]. Identifying which species are participating in bioremediation is difficult in a natural community. Through the use of a synthetic microbial community, the development of an artificial microbiome with functionally specific species is possible. Model systems for studying functional and structural characteristics can be found in these communities. Synthetic communities were formed by the co-culturing of two distinct microorganisms under precisely defined conditions, which were based on their interactions and functions [ 171 ]. The community’s dynamics and structure are determined by these variables; it is based on the discovery of bacterial processes and behaviors. Metabolism drives these patterns of microbial interaction, which in turn facilitates communication within communities [ 172 ]. Interactions between two microbial populations are social in nature (such as mutualism, competition, and cooperation). Cooperation is said to be a key factor in community structure and operation. Cooperation in community dynamics is influenced by the creation of synthetic communities [ 173 ] and it was found that modifying environmental conditions, such as deleting genes, could be used to engineer cooperation between two microbial strains. In addition to this, the synthetic community’s engineered microbial species have been examined for other patterns of interaction. Bioremediation strategies frequently make use of this type of engineered interaction [ 174 ]. It is possible to sustain the existence of microorganisms in a large population by using synthetic biology.
8. Advantages and Disadvantages
Due to the harm that pollutants exert on both humans and other living things, environmental pollution is a serious public health problem. The complete elimination of contaminants via chemical and physical methods of remediation is costly [ 175 ]. Additionally, both approaches may result in increased pollution and site disruption, which could have a detrimental effect on nearby humans and other biota. As a result, remediation techniques using chemicals and physical means are not regarded as eco-sustainable. Contrary to these techniques, bioremediation is the suggested solution to remove various persistent contaminants by relying on biological processes (mediated by various types of living organisms). However, all bioremediation techniques have their own advantages and disadvantages ( Figure 2 ) because they have their own specific applications [ 176 , 177 ].

Advantages and disadvantages of bioremediation.
9. Future Perspectives and Conclusions
Omics has gained prominence in the field of microbial remediation of the pulp and paper industry, textile industry, food industry, dairy industry, wood industry, fisheries, water and soil treatment industry, solid waste remediation, heavy metal pollution remediation, and hydrocarbon remediation. In order to better understand degradative pathways, bioremediation data must be mined, and new algorithms can be used to fit these data into simulation and numerical modeling with ease along with data assemblage, repositioning, exploration, and transmission, which necessitate standard protocols. Bioremediation processes may be better understood if new biomarkers are studied. Combining all the omics data with genetically-engineered tools could provide a comprehensive picture of the microbial remediation process. The role of phytoremediation in reducing environmental pollution can also be studied. The phytoremediation process has a number of advantages over other remediation strategies, including lower costs, greater public acceptance, and increased pollution degradation capacity [ 178 ]. Groundwater and air pollution, along with toxic waste generation as a by-product of semiconductor manufacturing, are problems for the environment; some examples include glycol ethers, hydrochloric acid (HCl), xylene, hydrogen fluoride (HF), and methanol [ 179 ]. In the case of pharmaceuticals that are designed to be long-lasting or even non-degradable, they pose a unique threat to the environment. The pharmaceutical pollutants are environmentally persistent substances. Trace amounts of pharmaceutical ingredients, such as birth control pills, anti-epileptics, pain relievers, and antidepressant medications, are found in many urban and rural sources of groundwater [ 180 ]. While operating, solar power generation facilities produce less greenhouse gas emissions, including air pollutants such as carbon monoxide, volatile organic compounds, nitrogen oxides, and carbon dioxide, than conventional fossil fuel-based power generation facilities [ 181 ]. Genetically-engineered plants can be able to bioremediate specific pollutants through discovered metabolic processes, enzymes, genes, or operons [ 182 ]. Although genomics, metabolomics, and proteomics in bioremediation aid in the exploration of possible solutions to specific pollutants, identifying and comparing gene and protein sequences that are effective at removing contaminants is the next step in bioremediation research. GMOs can clean up a wide range of waste effluents and polluted land [ 183 ]. When used in conjunction with other physical and chemical methods, bioremediation can provide a comprehensive approach toward removing pollution from the environment. Since it appears to be a long-term solution, there is a need for additional research in this area.
Acknowledgments
The author M.T. grateful to the Biotechnology Program, Department of Biochemistry, Dr. Rammanohar Lohia Avadh University, Ayodhya, India, for providing us with the research environment.
Funding Statement
This research received no external funding.
Author Contributions
Conceptualization, M.T., M.S., K.S. and B.S.I.; Writing—original draft preparation, S.B., D.G. and B.V.T.; Writing—review and editing, M.S., M.T., K.S. and B.V.T.; visualization, S.B. and D.G.; supervision, M.T., M.S., K.S. and B.S.I. All authors have read and agreed to the published version of the manuscript.
Institutional Review Board Statement
Not applicable.
Informed Consent Statement
Data availability statement, conflicts of interest.
The authors declare no conflict of interest.
Publisher’s Note: MDPI stays neutral with regard to jurisdictional claims in published maps and institutional affiliations.
Advertisement
Microbial remediation of oil-contaminated shorelines: a review
- Review Article
- Published: 12 August 2023
- Volume 30 , pages 93491–93518, ( 2023 )
Cite this article
- Xiaoli Dai ORCID: orcid.org/0000-0002-7109-7511 1 ,
- Jing Lv 2 ,
- Pengcheng Fu 3 &
- Shaohui Guo 2
671 Accesses
4 Citations
Explore all metrics
Frequent marine oil spills have led to increasingly serious oil pollution along shorelines. Microbial remediation has become a research hotspot of intertidal oil pollution remediation because of its high efficiency, low cost, environmental friendliness, and simple operation. Many microorganisms are able to convert oil pollutants into non-toxic substances through their growth and metabolism. Microorganisms use enzymes’ catalytic activities to degrade oil pollutants. However, microbial remediation efficiency is affected by the properties of the oil pollutants, microbial community, and environmental conditions. Feasible field microbial remediation technologies for oil spill pollution in the shorelines mainly include the addition of high-efficiency oil degrading bacteria (immobilized bacteria), nutrients, biosurfactants, and enzymes. Limitations to the field application of microbial remediation technology mainly include slow start-up, rapid failure, long remediation time, and uncontrolled environmental impact. Improving the environmental adaptability of microbial remediation technology and developing sustainable microbial remediation technology will be the focus of future research. The feasibility of microbial remediation techniques should also be evaluated comprehensively.
This is a preview of subscription content, log in via an institution to check access.
Access this article
Price includes VAT (Russian Federation)
Instant access to the full article PDF.
Rent this article via DeepDyve
Institutional subscriptions
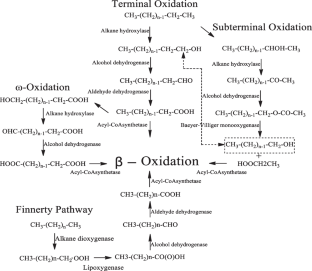
Similar content being viewed by others
Bioremediations for Oil Spills by Utilizing Microbes
Microbes and Petroleum Bioremediation
Microbial Remediation of Organometals and Oil Hydrocarbons in the Marine Environment
Data availability.
Not applicable.
Agarwal A, Liu Y (2015) Remediation technologies for oil-contaminated sediments. Mar. Pollut. Bull 101(2):483–490
Article CAS Google Scholar
Abou Khalil C, Fortin N, Prince RC, Greer CW, Lee K, Boufadel MC (2021a) Crude oil biodegradation in upper and supratidal seashores. J Hazard Mater 416:125919. https://doi.org/10.1016/j.jhazmat.2021.125919
Abou Khalil C, Prince RC, Greer CW, Lee K, Boufadel MC (2022) Bioremediation of petroleum hydrocarbons in the upper parts of sandy beaches. Environ Sci Technol 56(12):8124–8131. https://doi.org/10.1021/acs.est.2c01338
Abou Khalil C, Fortin N, Wasserscheid J, Prince RC, Greer CW, Lee K, Boufadel MC (2023) Microbial responses to increased salinity in oiled upper tidal shorelines. Int Biodeter Biodegr 181:105603. https://doi.org/10.1016/j.ibiod.2023.105603
Abbasian F, Lockington R, Mallavarapu M, Naidu R (2015) A comprehensive review of aliphatic hydrocarbon biodegradation by bacteria. Appl Biochem Biotechnol 176(3):670–699. https://doi.org/10.1007/s12010-015-1603-5
Abou Khalil C, Prince VL, Prince RC, Greer CW, Lee K, Zhang B, Boufadel MC (2021b) Occurrence and biodegradation of hydrocarbons at high salinities. Sci Total Environ 762:143165. https://doi.org/10.1016/j.scitotenv.2020.143165
Achuba FI, Okoh PN (2014) Effect of petroleum products on soil catalase and dehydrogenase activities. Open J Soil Sci 04(12):399–406. https://doi.org/10.4236/ojss.2014.412040
Article Google Scholar
Acosta-Gonzalez A, Marques S (2016) Bacterial diversity in oil-polluted marine coastal sediments. Curr Opin Biotechnol 38:24–32. https://doi.org/10.1016/j.copbio.2015.12.010
Aislabie J, Saul DJ, Foght JM (2006) Bioremediation of hydrocarbon-contaminated polar soils. Extremophiles 10(3):171–179. https://doi.org/10.1007/s00792-005-0498-4
Al-Awadhi H, Sulaiman RH, Mahmoud HM, Radwan SS (2007) Alkaliphilic and halophilic hydrocarbon-utilizing bacteria from Kuwaiti coasts of the Arabian Gulf. Appl Microbiol Biotechnol 77(1):183–186. https://doi.org/10.1007/s00253-007-1127-1
Al-Hawash AB, Dragh MA, Li S, Alhujaily A, Abbood HA, Zhang XY, Ma FY (2018) Principles of microbial degradation of petroleum hydrocarbons in the environment. Egypt J Aquat Res 44(2):71–76. https://doi.org/10.1016/j.ejar.2018.06.001
Alegbeleye OO, Opeolu BO, Jackson V (2017a) Bioremediation of polycyclic aromatic hydrocarbon (PAH) compounds: (acenaphthene and fluorene) in water using indigenous bacterial species isolated from the Diep and Plankenburg rivers, Western Cape, South Africa. Braz J Microbiol 48(2):314–325. https://doi.org/10.1016/j.bjm.2016.07.027
Alegbeleye OO, Opeolu BO, Jackson VA (2017b) Polycyclic aromatic hydrocarbons: a critical review of environmental occurrence and bioremediation. Environ Manage 60(4):758–783. https://doi.org/10.1007/s00267-017-0896-2
Arulazhagan P, Al-Shekri K, Huda Q, Godon JJ, Basahi JM, Jeyakumar D (2017) Biodegradation of polycyclic aromatic hydrocarbons by an acidophilic Stenotrophomonas maltophilia strain AJH1 isolated from a mineral mining site in Saudi Arabia. Extremophiles 21(1):163–174. https://doi.org/10.1007/s00792-016-0892-0
Arulazhagan P, Sivaraman C, Kumar SA, Aslam M, Banu JR (2014) Co-metabolic degradation of benzo (e) pyrene by halophilic bacterial consortium at different saline conditions. J Environ Biol 35(3):445
CAS Google Scholar
Austin B, Calomiris JJ, Walker JD, Colwell RR (1977) Numerical taxonomy and ecology of petroleum-degrading bacteria. Appl Environ Microbiol 34(1):60–68. https://doi.org/10.1128/aem.34.1.60-68.1977
Ayotamuno MJ, Kogbara RB, Ogaji SOT, Probert SD (2006) Bioremediation of a crude-oil polluted agricultural-soil at Port Harcourt, Nigeria. Appl Energy 83(11):1249–1257. https://doi.org/10.1016/j.apenergy.2006.01.003
Azizan NH, Abdul Rahim MS, Abidin ZAZ, Sharif MF, Chowdhury AJK (2020) Screening of biodegradation potential for n-alkanes and polycyclic aromatic hydrocarbon among isolates from the north-western tip of Pahang. Desalin Water Treat 191:207–212. https://doi.org/10.5004/dwt.2020.25304
Bacosa HP, Thyng KM, Plunkett S, Erdner DL, Liu Z (2016) The tarballs on Texas beaches following the 2014 Texas City “Y” Spill: modeling, chemical, and microbiological studies. Mar Pollut Bull 109(1):236–244. https://doi.org/10.1016/j.marpolbul.2016.05.076
Bacosa HP, Erdner DL, Liu Z (2015) Differentiating the roles of photooxidation and biodegradation in the weathering of Light Louisiana Sweet crude oil in surface water from the Deepwater Horizon site. Mar. Pollut. Bull 95(1):265–272
Barbier EB, Hacker SD, Kennedy C, Koch EW, Stier AC, Silliman BR (2011) The value of estuarine and coastal ecosystem services. Ecol Monogr 81:169–193. https://doi.org/10.1890/10-1510.1
Beam H, Perry J (1973) Co-metabolism as a factor in microbial degradation of cycloparaffinic hydrocarbons. Arch Microbiol 91(1):87–90. https://doi.org/10.1007/BF00409542
Bejarano AC, Michel J (2016) Oil spills and their impacts on sand beach invertebrate communities: a literature review. Environ Pollut 218:709–722. https://doi.org/10.1016/j.envpol.2016.07.065
Ben SO, Goni-Urriza MS, El Bour M, Dellali M, Aissa P, Duran R (2008) Characterization of aerobic polycyclic aromatic hydrocarbon-degrading bacteria from Bizerte lagoon sediments, Tunisia. J Appl Microbiol 104(4):987–997. https://doi.org/10.1111/j.1365-2672.2007.03621.x
Binazadeh M, Karimi IA, Li Z (2009) Fast biodegradation of long chain n-alkanes and crude oil at high concentrations with Rhodococcus sp. Moj-3449. Enzyme Microb Technol 45(3):195–202. https://doi.org/10.1016/j.enzmictec.2009.06.001
Boufadel M, Geng XL, An CJ, Owens E, Chen Z, Lee K, Taylor E, Prince RC (2019) A review on the factors affecting the deposition, retention, and biodegradation of oil stranded on beaches and guidelines for designing laboratory experiments. Curr Pollut Rep 5(4):407–423. https://doi.org/10.1007/s40726-019-00129-0
Brown LM, Gunasekera TS, Ruiz ON (2017) Draft genome sequence of Nocardioides Iuteus strain BAFB, an alkane-degrading bacterium isolated from JP-7-polluted soil. Genome Announc 5(4):1–2. https://doi.org/10.1128/genomeA.01529-16
Brown LM, Gunasekera TS, Striebich RC, Ruiz ON (2016) Draft genome sequence of Gordonia sihwensis strain 9, a branched alkane-degrading bacterium. Genome Announc 4(3). https://doi.org/10.1128/genomeA.00622-16
Brzeszcz J, Kaszycki P (2018) Aerobic bacteria degrading both n-alkanes and aromatic hydrocarbons: an undervalued strategy for metabolic diversity and flexibility. Biodegradation 29(4):359–407. https://doi.org/10.1007/s10532-018-9837-x
Bragg JR, Prince RC, Harner EJ, Atlas RM (1994) Effectiveness of bioremediation for the Exxon Valdez oil spill. Nature 368:413–418. https://doi.org/10.1038/368413a0
Conan G (1982) The long-term effects of the Amoco Cadiz oil spill. Phil. Trans. R. Soc. Lond. B 297(1087):323–333. https://doi.org/10.1098/rstb.1982.0045
Curl, H., Barton, K., Harris, L. (1992). Oil spill case histories, 1967-1991: Summaries of significant US and international spills. Final report (No. PB-93-144517/XAB; HMRAD-92-11). National Ocean Service, Seattle, WA (United States). Hazardous Materials Response and Assessment Div.
Callaghan AV (2013) Enzymes involved in the anaerobic oxidation of n-alkanes: from methane to long-chain paraffins. Front Microbiol 4(89):1–9. https://doi.org/10.3389/fmicb.2013.00089
Cebron A, Norini MP, Beguiristain T, Leyval C (2008) Real-time PCR quantification of PAH-ring hydroxylating dioxygenase (PAH-RHDalpha) genes from Gram positive and Gram negative bacteria in soil and sediment samples. J Microbiol Methods 73(2):148–159. https://doi.org/10.1016/j.mimet.2008.01.009
Chaıneaua CH, Morelb UJ, Duponta J, Bury E, Oudot J (1999) Comparison of the fuel oil biodegradation potential of hydrocarbon-assimilating microorganisms isolated from a temperate agricultural soil. Sci Total Environ 227:237–247. https://doi.org/10.1016/S0048-9697(99)00033-9
Chakraborty R, Coates JD (2004) Anaerobic degradation of monoaromatic hydrocarbons. Appl Microbiol Biotechnol 64(4):437–446. https://doi.org/10.1007/s00253-003-1526-x
Chakraborty R, O'Connor SM, Chan E, Coates JD (2005) Anaerobic degradation of benzene, toluene, ethylbenzene, and xylene compounds by Dechloromonas strain RCB. Appl Environ Microbiol 71(12):8649–8655. https://doi.org/10.1128/AEM.71.12.8649-8655.2005
Chaudhary P, Sharma R, Singh SB, Nain L (2011) Bioremediation of PAH by Streptomyces sp. Bull Environ Contam Toxicol 86(3):268–271. https://doi.org/10.1007/s00128-011-0211-5
Chen SH, Aitken MD (1999) Salicylate Stimulates the degradation of high-molecular weight polycyclic aromatic hydrocarbons by Pseudomonas saccharophila P15. Environ Sci Technol 33(3):435–439. https://doi.org/10.1021/es9805730
Chung WK, King GM (2001) Isolation, characterization, and polyaromatic hydrocarbon degradation potential of aerobic bacteria from marine macrofaunal burrow sediments and description of Lutibacterium anuloederans gen. nov., sp. nov., and Cycloclasticus spirillensus sp. nov. Appl Environ Microbiol 67(12):5585–5592. https://doi.org/10.1128/AEM.67.12.5585-5592.2001
Challenger GE, Gmur S, Taylor E (2015) A review of Gulf of Mexico coastal marsh erosion studies following the 2010 Deepwater Horizon oil spill and comparison to over 4 years of shoreline loss data from Fall 2010 to Summer 2015. Mar Pollut Bull 164:111983. https://doi.org/10.1016/j.marpolbul.2021.111983
Cong Dang PD, Ayako S, Hisanori T, Hoang Nguyen DP, Xo Hoa D, Yoshie T (2016) Identification and biodegradation characteristics of oil-degrading bacteria from subtropical Iriomote Island, Japan, and tropical Con Dao Island, Vietnam. Tropics 25(4):147–159. https://doi.org/10.3759/tropics.MS16-01
Cravo-Laureau C, Matheron R, Joulian C, Cayol JL, Hirschler-Rea A (2004) Desulfatibacillum alkenivorans sp. nov., a novel n-alkene-degrading, sulfate-reducing bacterium, and emended description of the genus Desulfatibacillum. Int J Syst Evol Microbiol 54(Pt 5):1639–1642. https://doi.org/10.1099/ijs.0.63104-0
Daane LL, Harjono I, Barns SM, Launen LA, Palleron NJ, Haggblom MM (2002) PAH-degradation by Paenibacillus spp. and description of Paenibacillus naphthalenovorans sp. nov., a naphthalene-degrading bacterium from the rhizosphere of salt marsh plants. Int J Syst Evol Microbiol 52(Pt 1):131–139. https://doi.org/10.1099/00207713-52-1-131
Dhaka A, Chattopadhyay P (2021) A review on physical remediation techniques for treatment of marine oil spills. J Environ Manage 288:112428. https://doi.org/10.1016/j.jenvman.2021.112428
Daccò C, Girometta C, Asemoloye MD, Carpani G, Picco AM, Tosi S (2020) Key fungal degradation patterns, enzymes and their applications for the removal of aliphatic hydrocarbons in polluted soils: A review. Int. Biodeter. Biodegr 147:104866. https://doi.org/10.1016/j.ibiod.2019.104866
Dai X, Lv J, Yan G, Chen C, Guo S, Fu P (2020) Bioremediation of intertidal zones polluted by heavy oil spilling using immobilized laccase-bacteria consortium. Bioresour. Technol 309:123305. https://doi.org/10.1016/j.biortech.2020.123305
Dai X, Lv J, Wei W, Guo S (2022) Bioremediation of heavy oil contaminated intertidal zones by immobilized bacterial consortium. Process Safety and Environmental Protection 158:70–78
Dai X, Yan G, Guo S (2017) Characterization of Dietzia cercidiphylli C-1 isolated from extra-heavy oil contaminated soil. Rsc Advances 7(32):19486–19491
Duke NC (2016) Oil spill impacts on mangroves: recommendations for operational planning and action based on a global review. Mar Pollut Bull 109:700–715. https://doi.org/10.1016/j.marpolbul.2016.06.082
Deng MC, Li J, Liang FR, Yi M, Xu XM, Yuan JP, Peng J, Wu CF, Wang JH (2014) Isolation and characterization of a novel hydrocarbon-degrading bacterium Achromobacter sp. HZ01 from the crude oil-contaminated seawater at the Daya Bay, southern China. Mar Pollut Bull 83(1):79–86. https://doi.org/10.1016/j.marpolbul.2014.04.018
Díaz, E. (2004). Bacterial degradation of aromatic pollutants: a paradigm of metabolic versatility.
Google Scholar
Dore SY, Clancy QE, Rylee SM, Kulpa CFJ (2003) Naphthalene-utilizing and mercury-resistant bacteria isolated from an acidic environment. Appl Microbiol Biotechnol 63(2):194–199. https://doi.org/10.1007/s00253-003-1378-4
Dubbels BL, Sayavedra-Soto LA, Bottomley PJ, Arp DJ (2009) Thauera butanivorans sp. nov., a C2-C9 alkane-oxidizing bacterium previously referred to as ‘Pseudomonas butanovora’. Int J Syst Evol Microbiol 59(Pt 7):1576–1578. https://doi.org/10.1099/ijs.0.000638-0
Dyksterhouse SE, Gray JP, Herwig RP, Lara JC, Staley JT (1995) Cycloclasticus pugetii gen. nov., sp. nov., an aromatic hydrocarbon-degrading bacterium from marine sediments. Int J Syst Bacteriol 45(1):116–123. https://doi.org/10.1099/00207713-45-1-116
Dave DAEG, Ghaly AE (2011) Remediation technologies for marine oil spills: A critical review and comparative analysis. Am. J. Environ. Sci 7(5):423
El-Gend NS (2006) Biodegradation potentials of dibenzothiophene by new bacteria isolated from hydrocarbon polluted soil in Egypt. Biosci Biotechnol Res Asia 3:95–106
El-Naas MH, Acio JA, El Telib AE (2014) Aerobic biodegradation of BTEX: progresses and prospects. J Environ Chem Eng 2(2):1104–1122. https://doi.org/10.1016/j.jece.2014.04.009
Engelhardt MA, Daly K, Swannell RPJ, Head IM (2001) Isolation and characterization of a novel hydrocarbon-degrading, Gram-positive bacterium, isolated from intertidal beach sediment, and description of Planococcus alkanoclasticus sp. nov. J Appl Microbiol 90:237–247. https://doi.org/10.1046/j.1365-2672.2001.01241.x
Feitkenhauer H, Müller R, Märkl H (2003) Degradation of polycyclic aromatic hydrocarbons and long chain alkanes at 6070 C by Thermus and Bacillus spp. Biodegradation 14:367–372. https://doi.org/10.1023/A:1027357615649
Feng L, Wang W, Cheng J, Ren Y, Zhao G, Gao C, Tang Y, Liu X, Han W, Peng X, Liu R, Wang L (2007) Genome and proteome of long-chain alkane degrading Geobacillus thermodenitrificans NG80-2 isolated from a deep-subsurface oil reservoir. Proc Natl Acad Sci USA 104(13):5602–5607. https://doi.org/10.1073/pnas.0609650104
Fuentes S, Mendez V, Aguila P, Seeger M (2014) Bioremediation of petroleum hydrocarbons: catabolic genes, microbial communities, and applications. Appl Microbiol Biotechnol 98(11):4781–4794. https://doi.org/10.1007/s00253-014-5684-9
Funhoff EG, Bauer U, Garcia-Rubio I, Witholt B, van Beilen JB (2006) CYP153A6, a soluble P450 oxygenase catalyzing terminal-alkane hydroxylation. J Bacteriol 188(14):5220–5227. https://doi.org/10.1128/JB.00286-06
Gallego JR, Menndez-Vega D, Gonzlez-Rojas E, Snchez J, Garcia-Martnez MJ, Llamas JF (2006) Oleophilic fertilizers and bioremediation: a new perspective. In: Modern multidisciplinary applied microbiology. Wiley-VCH Verlag GmbH & Co. KGaA, Weinheim, Germany, pp 551–555. https://doi.org/10.1002/9783527611904.ch97
Chapter Google Scholar
Gallo G, Piccolo LL, Renzone G, Rosa RL, Scaloni A, Quatrini P, Puglia AM (2012) Differential proteomic analysis of an engineered Streptomyces coelicolor strain reveals metabolic pathways supporting growth on n-hexadecane. Appl Microbiol Biotechnol 94:1289–1301. https://doi.org/10.1007/s00253-012-4046-8
Gao Y, Yu XZ, Wu SC, Cheung KC, Tam NF, Qian PY, Wong MH (2006) Interactions of rice (Oryza sativa L.) and PAH-degrading bacteria (Acinetobacter sp.) on enhanced dissipation of spiked phenanthrene and pyrene in waterlogged soil. Sci Total Environ 372(1):1–11. https://doi.org/10.1016/j.scitotenv.2006.09.029
Garcia-Olivares A, Aguero A, Haupt BJ, Marcos MJ, Villar MV, de Pablos JL (2017) A system of containment to prevent oil spills from sunken tankers. Sci Total Environ 593–594:242–252. https://doi.org/10.1016/j.scitotenv.2017.03.152
Guzman HM, Kaiser S, Weil E (2020) Assessing the long-term effects of a catastrophic oil spill on subtidal coral reef communities off the Caribbean coast of Panama (1985–2017). Mar. Biodivers 50:28
Geng XL, Boufadel MC, Jackson L (2016) Evidence of salt accumulation in beach intertidal zone due to evaporation. Sci Rep 6:31486. https://doi.org/10.1038/srep31486
Geng XL, Khalil CA, Prince RC, Lee K, An CJ, Boufadel MC (2021) Hypersaline pore water in Gulf of Mexico beaches prevented efficient biodegradation of Deepwater Horizon beached oil. Environ Sci Technol 55(20):13792–13801. https://doi.org/10.1021/acs.est.1c02760
Gaur VK, Gupta S, Pandey A (2021) Evolution in mitigation approaches for petroleum oil-polluted environment: recent advances and future directions. Environ Sci Pollut Res Int 29:61821–61837
Ghosal D, Dutta A, Chakraborty J, Basu S, Dutta TK (2013) Characterization of the metabolic pathway involved in assimilation of acenaphthene in Acinetobacter sp. strain AGAT-W. Res Microbiol 164(2):155–163. https://doi.org/10.1007/s11356-021-16047-y
Ghosal D, Ghosh S, Dutta TK, Ahn Y (2016) Current state of knowledge in microbial degradation of polycyclic aromatic hydrocarbons (PAHs): a review. Front Microbiol 7:1369. https://doi.org/10.3389/fmicb.2016.01369
Ghosh S, Chakraborty S (2020) Production of polyhydroxyalkanoates (PHA) from aerobic granules of refinery sludge and Micrococcus aloeverae strain SG002 cultivated in oily wastewater. Int Biodeterior Biodegr 155:105091. https://doi.org/10.1016/j.ibiod.2020.105091
Gibtan A, Park K, Woo M, Shin JK, Lee DW, Sohn JH, Song M, Roh SW, Lee SJ, Lee HS (2017) Diversity of extremely halophilic archaeal and bacterial communities from commercial salts. Front Microbiol 8:799. https://doi.org/10.3389/fmicb.2017.00799
Golyshin PN (2002) Oleiphilaceae fam. nov., to include Oleiphilus messinensis gen. nov., sp. nov., a novel marine bacterium that obligately utilizes hydrocarbons. Int J Syst Evol Micr 52(3):901–911. https://doi.org/10.1099/00207713-52-3-901
Gongora E, Chen YJ, Ellis M, Okshevsky M, Whyte L (2022) Hydrocarbon bioremediation on Arctic shorelines: historic perspective and roadway to the future. Environ. Pollut 305:119247. https://doi.org/10.1016/j.envpol.2022.119247
Guo CL, Dang Z, Wong Y, Tam NF (2010) Biodegradation ability and dioxgenase genes of PAH-degrading Sphingomonas and Mycobacterium strains isolated from mangrove sediments. Int Biodeter Biodegr 64(6):419–426. https://doi.org/10.1016/j.ibiod.2010.04.008
Gurav R, Lyu HH, Ma JL, Tang JC, Liu QL, Zhang HR (2017) Degradation of n-alkanes and PAHs from the heavy crude oil using salt-tolerant bacterial consortia and analysis of their catabolic genes. Environ Sci Pollut Res 24(12):11392–11403. https://doi.org/10.1007/s11356-017-8446-2
Habe H, Omori T (2003) Genetics of polycyclic aromatic hydrocarbon metabolism in diverse aerobic bacteria. Biosci Biotech Bioch 67(2):225–243. https://doi.org/10.1271/bbb.67.225
Hoff RZ (1993) Bioremediation: an overview of its development and use for oil spill cleanup. Mar Pollut Bull 26(9):476–481. https://doi.org/10.1016/0025-326X(93)90463-T
Hoff RZ (1992) A summary of bioremediation applications observed at marine oil spill. Report HMRB 91-2. Hazardous Materials Response and Assessment Division Seattle, National Oceanic and Atmospheric Administration, Washington.
Hajieghrari M, Hejazi P (2020) Enhanced biodegradation of n-Hexadecane in solid-phase of soil by employing immobilized Pseudomonas Aeruginosa on size-optimized coconut fibers. J Hazard Mater 389:122134. https://doi.org/10.1016/j.jhazmat.2020.122134
Hamann C, Hegemann J, Hildebrandt A (1999) Detection of polycyclic aromatic hydrocarbon degradation genes in different soil bacteria by polymerase chain reaction and DNA hybridization. FEMS Microbiol Lett 173:255–263. https://doi.org/10.1111/j.1574-6968.1999.tb13510.x
Hassanshahian M, Ahmadinejad M, Tebyanian H, Kariminik A (2013) Isolation and characterization of alkane degrading bacteria from petroleum reservoir waste water in Iran (Kerman and Tehran provenances). Mar Pollut Bull 73(1):300–305. https://doi.org/10.1016/j.marpolbul.2013.05.002
Head IM, Jones DM, Roling WF (2006) Marine microorganisms make a meal of oil. Nat Rev Microbiol 4(3):173–182. https://doi.org/10.1038/nrmicro1348
Hedlund BP, Geiselbrecht AD, Bair TJ, Staley JT (1999) Polycyclic aromatic hydrocarbon degradation by a new marine bacterium, Neptunomonas naphthovorans gen. nov., sp. nov. Appl Environ Microbiol 65:251–259. https://doi.org/10.1128/AEM.65.1.251-259.1999
Hirano S, Kitauchi F, Haruki M, Imanaka T, Morikawa M, Kanaya S (2004) Isolation and characterization of Xanthobacter polyaromaticivorans sp. nov. 127W that degrades polycyclic and heterocyclic aromatic compounds under extremely low oxygen conditions. Biosci Biotechnol Biochem 68(3):557–564. https://doi.org/10.1271/bbb.68.557
Horvath RS (1972) Microbial co-metabolism and the degradation of organic compounds in nature. Bacteriol Rev 36(2):146. https://doi.org/10.1128/br.36.2.146-155.1972
Hua X, Wu Z, Zhang H, Lu D, Wang M, Liu Y, Liu Z (2010) Degradation of hexadecane by Enterobacter cloacae strain TU that secretes an exopolysaccharide as a bioemulsifier. Chemosphere 80(8):951–956. https://doi.org/10.1016/j.chemosphere.2010.05.002
Ilori MO, Amobi CJ, Odocha AC (2005) Factors affecting biosurfactant production by oil degrading Aeromonas spp. isolated from a tropical environment. Chemosphere 61(7):985–992. https://doi.org/10.1016/j.chemosphere.2005.03.066
ITOPF (2016) The international tanker owners pollution federation limited oil tanker spill statistics 2015. Retrieved March 16:2016 https://www.itopf.org/knowledge-resources/documents-guides/
Iturbe-Espinoza P, Bonte M, Gundlach E, Brandt BW, Braster M, van Spanning RJM (2022) Adaptive changes of sediment microbial communities associated with cleanup of oil spills in Nigerian mangrove forests. Mar Pollut Bull 2022(176):113406. https://doi.org/10.1016/j.marpolbul.2022.113406
Ji W, Abou-Khalil C, Jayalakshmamma MP, Boufadel MC, Lee K (2023) Post-formation of oil particle aggregates: breakup and biodegradation. Environ Sci Technol 57(6):2341–2350. https://doi.org/10.1021/acs.est.2c05866
Jaekel U, Zedelius J, Wilkes H, Musat F (2015) Anaerobic degradation of cyclohexane by sulfate-reducing bacteria from hydrocarbon-contaminated marine sediments. Front Microbiol 6:116
Jeon CO, Park W, Ghiorse WC, Madsen EL (2004) Polaromonas naphthalenivorans sp. nov., a naphthalene-degrading bacterium from naphthalene-contaminated sediment. Int J Syst Evol Microbiol 54(Pt 1):93–97. https://doi.org/10.3389/fmicb.2015.00116
Jin HM, Kim JM, Lee HJ, Madsen EL, Jeon CO (2012) Alteromonas as a key agent of polycyclic aromatic hydrocarbon biodegradation in crude oil-contaminated coastal sediment. Environ Sci Technol 46(14):7731–7740. https://doi.org/10.1021/es3018545
Juhasz AL, Naidu R (2000) Bioremediation of high molecular weight polycyclic aromatic hydrocarbons: a review of the microbial degradation of benzo[a ]pyrene. Int Biodeterior Biodegr 45:57–88. https://doi.org/10.1016/S0964-8305(00)00052-4
Kaczorek E (2012) Effect of external addition of rhamnolipids biosurfactant on the modification of gram positive and gram negative bacteria cell surfaces during biodegradation of hydrocarbon fuel contamination. Pol J of Environ Stud 21(4):901–909
Kadri T, Rouissi T, Kaur Brar S, Cledon M, Sarma S, Verma M (2017) Biodegradation of polycyclic aromatic hydrocarbons (PAHs) by fungal enzymes: a review. J Environ Sci (China) 51:52–74. https://doi.org/10.1016/j.jes.2016.08.023
Kaplan CW, Kitts CL (2004) Bacterial succession in a petroleum land treatment unit. Appl Environ Microbiol 70(3):1777–1786. https://doi.org/10.1128/AEM.70.3.1777-1786.2004
Karlapudi AP, Venkateswarulu TC, Tammineedi J, Kanumuri L, Ravuru BK, Dirisala VR, Kodali VP (2018) Role of biosurfactants in bioremediation of oil pollution-a review. Petroleum 4(3):241–249. https://doi.org/10.1016/j.petlm.2018.03.007
Kato C, lnoue A, Horikoshi K (1996) Isolating and characterizing deep-sea marine microorganisms. Trends Biotechnol 14: 6–12. https://doi.org/10.1016/0167-7799(96)80907-3
Khan MAI, Biswas B, Smith E, Mahmud SA, Hasan NA, Khan MAW, Naidu R, Megharaj M (2018) Microbial diversity changes with rhizosphere and hydrocarbons in contrasting soils. Ecotoxicol Environ Saf 156:434–442. https://doi.org/10.1016/j.ecoenv.2018.03.006
Khara P, Roy M, Chakraborty J, Ghosal D, Dutta TK (2014) Functional characterization of diverse ring-hydroxylating oxygenases and induction of complex aromatic catabolic gene clusters in Sphingobium sp. PNB. FEBS Open Bio 4:290–300. https://doi.org/10.1016/j.fob.2014.03.001
Kim JG, Kim SH, Yoon JH, Lee PC (2013) Carotenoid production from n-alkanes with a broad range of chain lengths by the novel species Gordonia ajoucoccus A2 T . Appl Microbiol Biotechnol 98:3759–3768. https://doi.org/10.1007/s00253-014-5516-y
Kim SJ, Park SJ, Jung M, Kim JG, Min UG, Hong HJ, Rhee SK (2014) Draft genome sequence of an aromatic compound-degrading bacterium, Desulfobacula sp. TS, belonging to the Deltaproteobacteria. FEMS Microbiol Lett 360(1):9–12. https://doi.org/10.1111/1574-6968.12591
Kleindienst S, Herbst FA, Stagars M, von Netzer F, von Bergen M, Seifert J, Peplies J, Amann R, Musat F, Lueders T, Knittel K (2014) Diverse sulfate-reducing bacteria of the Desulfosarcina/Desulfococcus clade are the key alkane degraders at marine seeps. ISME J 8(10):2029–2044. https://doi.org/10.1038/ismej.2014.51
Kleindienst S, Paul JH, Joye SB (2015) Using dispersants after oil spills: impacts on the composition and activity of microbial communities. Nat Rev Microbiol 13(6):388–396. https://doi.org/10.1038/nrmicro3452
Kniemeyer O, Musat F, Sievert SM, Knittel K, Wilkes H, Blumenberg M, Michaelis W, Classen A, Bolm C, Joye SB, Widdel F (2007) Anaerobic oxidation of short-chain hydrocarbons by marine sulphate-reducing bacteria. Nature 449(7164):898–901. https://doi.org/10.1038/nature06200
Kostichka K, Thomas SM, Gibson KJ, Nagarajan V, Cheng Q (2001) Cloning and characterization of a gene cluster for cyclododecanone oxidation in Rhodococcus ruber SC1. J Bacteriol 183(21):6478–6486. https://doi.org/10.1128/JB.183.21.6478-6486.2001
Kotani T, Kawashima Y, Yurimoto H, Kato N, Sakai Y (2006) Gene structure and regulation of alkane monooxygenases in propane-utilizing Mycobacterium sp. TY-6 and Pseudonocardia sp. TY-7. J. Biosci. Bioeng. 102(3):184–192. https://doi.org/10.1263/jbb.102.184
Kweon O, Kim SJ, Baek S, Chae JC, Adjei MD, Baek DH, Kim YC, Cerniglia CE (2008) A new classification system for bacterial Rieske non-heme iron aromatic ring-hydroxylating oxygenases. BMC Biochem 9:11. https://doi.org/10.1186/1471-2091-9-11
Lal B, Khanna S (1996) Degradation of crude oil by Acinetobacter calcoaceticus and Alcaligenes odorans. J. Appl. Microbiol 81(4):355–362
Lawniczak L, Wozniak-Karczewska M, Loibner AP, Heipieper HJ, Chrzanowski L (2020) Microbial degradation of hydrocarbons-basic principles for bioremediation: a review. Molecules 25(4). https://doi.org/10.3390/molecules25040856
Le TN, Mikolasch A, Awe S, Sheikhany H, Klenk HP, Schauer F (2010) Oxidation of aliphatic, branched chain, and aromatic hydrocarbons by Nocardia cyriacigeorgica isolated from oil-polluted sand samples collected in the Saudi Arabian Desert. J Basic Microbiol 50(3):241–253. https://doi.org/10.1002/jobm.200900358
Lee K, Boufadel M, Chen B, Foght J, Hodson P, Swanson S, Venosa A (2015) Expert panel report on the behaviour and environmental impacts of crude oil released into aqueous environments. Royal Society of Canada, Ottawa, 488.
Li X, Zhao L, Adam M (2016) Biodegradation of marine crude oil pollution using a salt-tolerant bacterial consortium isolated from Bohai Bay, China. Mar Pollut Bull 105(1):43–50. https://doi.org/10.1016/j.marpolbul.2016.02.073
Lim MW, Lau EV, Poh PE (2016) A comprehensive guide of remediation technologies for oil contaminated soil - present works and future directions. Mar Pollut Bull 109(1):14–45. https://doi.org/10.1016/j.marpolbul.2016.04.023
Liu A, Garcia-Dominguez E, Rhine ED, Young LY (2004) A novel arsenate respiring isolate that can utilize aromatic substrates. FEMS Microbiol Ecol 48(3):323–332. https://doi.org/10.1016/j.femsec.2004.02.008
Liu C, Shao Z (2005) Alcanivorax dieselolei sp. nov., a novel alkane-degrading bacterium isolated from sea water and deep-sea sediment. Int. J. Syst. Evol. Microbiol. 55(Pt 3):1181–1186. https://doi.org/10.1099/ijs.0.63443-0
Li H, Boufadel MC (2010) Long-term persistence of oil from the Exxon Valdez spill in two-layer beaches. Nat Geosci 3(2):96–99. https://doi.org/10.1038/ngeo749
Liu C, Wang W, Wu Y, Zhou Z, Lai Q, Shao Z (2011) Multiple alkane hydroxylase systems in a marine alkane degrader, Alcanivorax dieselolei B-5. Environ. Microbiol 13(5):1168–1178. https://doi.org/10.1111/j.1462-2920.2010.02416.x
Liu ZF, Liu JQ, Zhu QZ, Wu W (2012) The weathering of oil after the Deepwater Horizon oil spill: insights from the chemical composition of the oil from the sea surface, salt marshes and sediments. Environ Res Lett 7:035302. https://doi.org/10.1088/1748-9326/7/3/035302
Liu Q, Tang J, Gao K, Gurav R, Giesy JP (2017a) Aerobic degradation of crude oil by microorganisms in soils from four geographic regions of China. Sci Rep 7(1):14856. https://doi.org/10.1038/s41598-017-14032-5
Liu Y, Zeng G, Zhong H, Wang Z, Liu Z, Cheng M, Liu G, Yang X, Liu S (2017b) Effect of rhamnolipid solubilization on hexadecane bioavailability: enhancement or reduction? J Hazard Mater 322(Pt B): 394–401. https://doi.org/10.1016/j.jhazmat.2016.10.025
Lovley DR, Lonergan DJ (1990) Anaerobic oxidation of toluene, phenol, and p-cresol by the dissimilatory iron-reducing organism, GS-15. Appl Environ Microbiol 56(6):1858–1864. https://doi.org/10.1128/aem.56.6.1858-1864.1990
Luo W, Zhao Y, Ding H, Lin X, Zheng H (2008) Co-metabolic degradation of bensulfuron-methyl in laboratory conditions. J Hazard Mater 158(1):208–214. https://doi.org/10.1016/j.jhazmat.2008.02.115
Luo W, Zhu X, Chen W, Duan Z, Wang L, Zhou Y (2014) Mechanisms and strategies of microbial cometabolism in the degradation of organic compounds - chlorinated ethylenes as the model. Water Sci Technol 69(10):1971–1983. https://doi.org/10.2166/wst.2014.108
Lv M, Luan X, Liao C, Wang D, Liu D, Zhang G, Jiang G, Chen L (2020) Human impacts on polycyclic aromatic hydrocarbon distribution in Chinese intertidal zones. Nat Sustain 3(10):878–884. https://doi.org/10.1038/s41893-020-0565-y
Michel J, Fegley SR, Dahlin JA, Wood C (2017) Oil spill response-related injuries on sand beaches: When shoreline treatment extends the impacts beyond the oil. Mar Ecol Prog Ser 576:203–218. https://doi.org/10.3354/meps11917
Ma YL, Lu W, Wan LL, Luo N (2015) Elucidation of fluoranthene degradative characteristics in a newly isolated Achromobacter xylosoxidans DN002. Appl Biochem Biotechnol 175(3):1294–1305. https://doi.org/10.1007/s12010-014-1347-7
Mahmoud GA, Bagy MMK (2018) Microbial degradation of petroleum hydrocarbons. Microbial Action on Hydrocarbons:299–320. https://doi.org/10.1007/978-981-13-1840-5_12
Martínez-Palou R, de Lourdes MM, Zapata-Rendón B, Mar-Juárez E, Bernal-Huicochea C, de la Cruz C-LJ, Aburto J (2011) Transportation of heavy and extra-heavy crude oil by pipeline: a review. J Pet Sci Eng 75(3-4):274–282. https://doi.org/10.1016/j.petrol.2010.11.020
Maiti A, Das S, Bhattacharyya N (2012) Bioremediation of high molecular weight polycyclic aromatic hydrocarbons by Bacillus thuringiensis strain NA2. J Sci 1:72–75
Mallick S, Chakraborty J, Dutta TK (2011) Role of oxygenases in guiding diverse metabolic pathways in the bacterial degradation of low-molecular-weight polycyclic aromatic hydrocarbons: a review. Crit Rev Microbiol 37(1):64–90. https://doi.org/10.3109/1040841X.2010.512268
Mallick S, Chatterjee S, Dutta TK (2007) A novel degradation pathway in the assimilation of phenanthrene by Staphylococcus sp. strain PN/Y via meta-cleavage of 2-hydroxy-1-naphthoic acid: formation of trans-2,3-dioxo-5-(2'-hydroxyphenyl)-pent-4-enoic acid. Microbiology (Reading) 153(Pt 7):2104–2115. https://doi.org/10.1099/mic.0.2006/004218-0
McGenity TJ (2014) Hydrocarbon biodegradation in intertidal wetland sediments. Curr Opin Biotechnol 27:46–54. https://doi.org/10.1016/j.copbio.2013.10.010
McGenity TJ (2019) Taxonomy, genomics and ecophysiology of hydrocarbon-degrading microbes. Hydrocarbon-Degrading Microbes 12
Meckenstock RU, Boll M, Mouttaki H, Koelschbach JS, Cunha Tarouco P, Weyrauch P, Dong X, Himmelberg AM (2016) Anaerobic degradation of benzene and polycyclic aromatic hydrocarbons. J Mol Microbiol Biotechnol 26(1–3):92–118. https://doi.org/10.1159/000441358
Meckenstock RU, Mouttaki H (2011) Anaerobic degradation of non-substituted aromatic hydrocarbons. Curr Opin Biotechnol 22(3):406–414. https://doi.org/10.1016/j.copbio.2011.02.009
Megharaj M, Ramakrishnan B, Venkateswarlu K, Sethunathan N, Naidu R (2011) Bioremediation approaches for organic pollutants: a critical perspective. Environ. Int 37(8):1362–1375. https://doi.org/10.1016/j.envint.2011.06.003
Mercer K, Trevors JT (2011) Remediation of oil spills in temperate and tropical coastal marine environments. The Environmentalist 31(3):338–347. https://doi.org/10.1007/s10669-011-9335-8
Meyer S, Moser R, Neef A, Stahl U, Kampfer P (1999) Differential detection of key enzymes of polyaromatic-hydrocarbon-degrading bacteria using PCR and gene probes. Microbiology 145(Pt 7):1731–1741. https://doi.org/10.1099/13500872-145-7-1731
Meyer S, Steinhart H (2000) Effects of heterocyclic PAHs (N S O) on the biodegradation of typical tar oil PAHs in a soil compost mixture. Chemosphere 40(4):359–367. https://doi.org/10.1016/S0045-6535(99)00237-4
Minerdi D, Sadeghi SJ, Di Nardo G, Rua F, Castrignanò S, Allegra P, Gilardi G (2015) CYP116B5: a new class VII catalytically self-sufficient cytochrome P450 from Acinetobacter radioresistens that enables growth on alkanes. Mol. Microbio 95(3):539–554. https://doi.org/10.1111/mmi.12883
Miralles G, Grossi V, Acquaviva M, Duran R, Claude Bertrand J, Cuny P (2007) Alkane biodegradation and dynamics of phylogenetic subgroups of sulfate-reducing bacteria in an anoxic coastal marine sediment artificially contaminated with oil. Chemosphere 68(7):1327–1334. https://doi.org/10.1016/j.chemosphere.2007.01.033
Mishra B, Varjani S, Agrawal DC, Mandal SK, Ngo HH, Taherzadeh MJ, Chang JS, You S, Guo W (2020) Engineering biocatalytic material for the remediation of pollutants: a comprehensive review. Environ. Technol. Innov 20:101063. https://doi.org/10.1016/j.eti.2020.101063
Mishra S, Singh SN (2012) Microbial degradation of n-hexadecane in mineral salt medium as mediated by degradative enzymes. Bioresour Technol 111:148–154. https://doi.org/10.1016/j.biortech.2012.02.049
Mohamad Shahimin MF, Foght JM, Siddique T (2016) Preferential methanogenic biodegradation of short-chain n-alkanes by microbial communities from two different oil sands tailings ponds. Sci Total Environ 553:250–257. https://doi.org/10.1016/j.scitotenv.2016.02.061
Moreno R, Rojo F (2019) Enzymes for aerobic degradation of alkanes in bacteria. Aerobic utilization of hydrocarbons, oils, and lipids, handbook of hydrocarbon and lipid microbiology:117–142
Muangchinda C, Pansri R, Wongwongsee W, Pinyakong O (2013) Assessment of polycyclic aromatic hydrocarbon biodegradation potential in mangrove sediment from Don Hoi Lot, Samut Songkram Province, Thailand. J Appl Microbiol 114(5):1311–1324. https://doi.org/10.1111/jam.12128
Muratova AY, Turkovskaya OV, Antonyuk LP, Makarov OE, Pozdnyakova LI, Ignatov VV (2005) Oil-oxidizing potential of associative Rhizobacteria of the genus Azospirillum. Microbiology 74(2):210–215. https://doi.org/10.1007/s11021-005-0053-4
Nhi-Cong LT, Lien DT, Gupta BS, Mai CTN, Ha HP, Nguyet NTM, Duan TH, Van Quyen D, Zaid HFM, Sankaran R, Show PL (2020) Enhanced degradation of diesel oil by using biofilms formed by indigenous purple photosynthetic bacteria from oil-contaminated coasts of vietnam on different carriers. Appl Biochem Biotechnol 191(1):313–330. https://doi.org/10.1007/s12010-019-03203-x
Nhi-Cong LT, Mikolasch A, Klenk HP, Schauer F (2009) Degradation of the multiple branched alkane 2,6,10,14-tetramethyl-pentadecane (pristane) in Rhodococcus ruber and Mycobacterium neoaurum. Int Biodeter Biodegr 63(2):201–207. https://doi.org/10.1016/j.ibiod.2008.09.002
Nie Y, Liang JL, Fang H, Tang YQ, Wu XL (2014) Characterization of a CYP153 alkane hydroxylase gene in a Gram-positive Dietzia sp. DQ12-45-1b and its “team role” with alkW1 in alkane degradation. Appl Microbiol Biotechnol 98(1):163–173. https://doi.org/10.1007/s00253-013-4821-1
Nopcharoenkul W, Netsakulnee P, Pinyakong O (2013) Diesel oil removal by immobilized Pseudoxanthomonas sp.RN402. Biodegradation 24:387–397. https://doi.org/10.1007/s10532-012-9596-z
Nwankwegu AS, Zhang L, Xie D, Onwosi CO, Muhammad WI, Odoh CK, Sam K, Idenyi JN (2022) Bioaugmentation as a green technology for hydrocarbon pollution remediation. Problems and prospects. J Environ Manage 304:114313. https://doi.org/10.1016/j.jenvman.2021.114313
Nzila A (2018) Biodegradation of high-molecular-weight polycyclic aromatic hydrocarbons under anaerobic conditions: overview of studies, proposed pathways and future perspectives. Environ Pollut 239:788–802
Obahiagbon KO, Amenaghawon AN, Agbonghae EO (2014) The effect of initial pH on the bioremediation of crude oil polluted water using a consortium of microbes. Pacific J Sci Technol 15(1). https://doi.org/10.1016/j.envpol.2018.04.074
Parales RE, Ditty JL, Harwood CS (2000) Toluene-degrading bacteria are chemotactic towards the environmental pollutants benzene, toluene, and trichloroethylene. Appl Environ Microbiol 66(9):4098–4104. https://doi.org/10.1128/AEM.66.9.4098-4104.2000
Partovinia A, Rasekh B (2018) Review of the immobilized microbial cell systems for bioremediation of petroleum hydrocarbons polluted environments. Crit Rev Environ Sci Technol 48(1):1–38. https://doi.org/10.1080/10643389.2018.1439652
Patel V, Cheturvedula S, Madamwar D (2012) Phenanthrene degradation by Pseudoxanthomonas sp. DMVP2 isolated from hydrocarbon contaminated sediment of Amlakhadi canal, Gujarat, India. J Hazard Mater 201–202:43–51. https://doi.org/10.1016/j.jhazmat.2011.11.002
Pei XH, Zhan XH, Wang SM, Lin YS, Zhou LX (2010) Effects of a biosurfactant and a synthetic surfactant on phenanthrene degradation by a sphingomonas strain. Pedosphere 20(6):771–779. https://doi.org/10.1016/S1002-0160(10)60067-7
Perry JJ (2015) Thermoleophilum. BMSAB:1–6
Pi Y, Xu N, Bao M, Li Y, Lv D, Sun P (2015) Bioremediation of the oil spill polluted marine intertidal zone and its toxicity effect on microalgae. Environ Sci-Proc Imp 17:877–885. https://doi.org/10.1016/S1002-0160(10)60067-7
Pilloni G, von Netzer F, Engel M, Lueders T (2011) Electron acceptor-dependent identification of key anaerobic toluene degraders at a tar-oil-contaminated aquifer by Pyro-SIP. FEMS Microbiol Ecol 78(1):165–175. https://doi.org/10.1111/j.1574-6941.2011.01083.x
Pleshakova YV, Belyakov AY, Deev DV (2019) Characteristics of hydrocarbon degradation by bacteria isolated from drill cuttings. Biology Bulletin 45(10):1174–1181. https://doi.org/10.1134/S1062359018100229
Prince RC (2005) The microbiology of marine oil spill bioremediation. Petroleum Microbiology:318–335. https://doi.org/10.1128/9781555817589.ch16
Prince RC, McFarlin KM, Butler JD, Febbo EJ, Wang FC, Nedwed TJ (2013) The primary biodegradation of dispersed crude oil in the sea. Chemosphere 90(2):521–526. https://doi.org/10.1016/j.chemosphere.2012.08.020
Prince RC, Bragg JR (1997) Shoreline bioremediation following the Exxon Valdez oil spill in Alaska. Bioremediat J 1(2):97–104 https://doi.org/10.1080/10889869709351324
Price ARG (1998) Impact of the 1991 Gulf War on the coastal environment and ecosystems: Current status and future prospects. Environ Int 24(1–2):91–96. https://doi.org/10.1016/S0160-4120(97)00124-4
Qi YB, Wang CY, Lv CY, Lun ZM, Zheng CG (2017) Removal capacities of polycyclic aromatic hydrocarbons (PAHs) by a newly isolated strain from oilfield produced water. Int J Environ Res Public Health 14(2). https://doi.org/10.3390/ijerph14020215
Rabus R, Boll M, Heider J, Meckenstock RU, Buckel W, Einsle O, Ermler U, Golding BT, Gunsalus RP, Kroneck PM, Kruger M, Lueders T, Martins BM, Musat F, Richnow HH, Schink B, Seifert J, Szaleniec M, Treude T et al (2016) Anaerobic microbial degradation of hydrocarbons: from enzymatic reactions to the environment. J Mol Microbiol Biotechnol 26(1–3):5–28. https://doi.org/10.1159/000443997
Rabalais S, Flint R (1983) Ixtoc-I effects on intertidal and subtidal infauna of south Texas Gulf beaches. Contrib Mar Sci 26:23–35 http://hdl.handle.net/1969.3/27416
Rabus R, Widdel F (1995) Anaerobic degradation of ethylbenzene and other aromatic hydrocarbons by new denitrifying bacteria. Arch Microbiot 163:96–103. https://doi.org/10.1007/BF00381782
Radwan SS, Al-Hasan RH, Mahmoud HM, Eliyas M (2007) Oil-utilizing bacteria associated with fish from the Arabian Gulf. J Appl Microbiol 103(6):2160–2167. https://doi.org/10.1111/j.1365-2672.2007.03454.x
Ramsay MA, Swannell RPJ, Shipton W, Duke NC (2000) Effect of bioremediation on the microbial community in oiled mangrove sediments. Mar Pollut Bull 41(7–12):413–419. https://doi.org/10.1016/S0025-326X(00)00137-5
Rojo F (2009) Degradation of alkanes by bacteria. Environ Microbiol 11(10):2477–2490. https://doi.org/10.1111/j.1462-2920.2009.01948.x
Ron EZ, Rosenberg E (2014) Enhanced bioremediation of oil spills in the sea. Curr Opin Biotechnol 27:191–194. https://doi.org/10.1016/j.copbio.2014.02.004
Ruan B, Wu P, Chen M, Lai X, Chen L, Yu L, Gong B, Kang C, Dang Z, Shi Z, Liu Z (2018) Immobilization of Sphingomonas sp. GY2B in polyvinyl alcohol-alginate-kaolin beads for efficient degradation of phenol against unfavorable environmental factors. Ecotoxicol Environ Saf 162:103–111. https://doi.org/10.1016/j.ecoenv.2018.06.058
Saito A, Iwabuchi T, Harayama S (2000) A novel phenanthrene dioxygenase from Nocardioides sp. strain KP7: expression in Escherichia coli. J Bacteriol 182:2134–2144. https://doi.org/10.1128/JB.182.8.2134-2141.2000
Sakai Y, Maeng JH, Kubota S, Tani A, Tani Y, Kato N (1996) A non-conventional dissimilation pathway for long chain n-alkanes in Acinetobacter sp. M-1 that starts with a dioxygenase reaction. J Ferment Bioeng 8(4):286–291. https://doi.org/10.1016/0922-338X(96)80578-2
Santiago MB, Moraes TDS, Massuco JE, Silva LO, Lucarini R, da Silva DF, Vieira TM, Crotti AEM, Martins CHG (2018) In vitro evaluation of essential oils for potential antibacterial effects against Xylella fastidiosa. J Phytopathol 166(11–12):790–798. https://doi.org/10.1111/jph.12762
Sarma PM, Duraja P, Deshpande S, Lal B (2010) Degradation of pyrene by an enteric bacterium, Leclercia adecarboxylata PS4040. Biodegradation 21(1):59–69. https://doi.org/10.1007/s10532-009-9281-z
Scheps D, Malca SH, Hoffmann H, Nestl BM, Hauer B (2011) Regioselective omega-hydroxylation of medium-chain n-alkanes and primary alcohols by CYP153 enzymes from Mycobacterium marinum and Polaromonas sp. strain JS666. Org Biomol Chem 9(19):6727–6733. https://doi.org/10.1039/C1OB05565H
Schneiker S, Martins dos Santos VA, Bartels D, Bekel T, Brecht M, Buhrmester J, Chernikova TN, Denaro R, Ferrer M, Gertler C, Goesmann A, Golyshina OV, Kaminski F, Khachane AN, Lang S, Linke B, McHardy AC, Meyer F, Nechitaylo T et al (2006) Genome sequence of the ubiquitous hydrocarbon-degrading marine bacterium Alcanivorax borkumensis. Nat Biotechnol 24(8):997–1004. https://doi.org/10.1038/nbt1232
Sekine M, Tanikawa S, Omata S, Saito M, Fujisawa T, Tsukatani N, Tajima T, Sekigawa T, Kosugi H, Matsuo Y, Nishiko R, Imamura K, Ito M, Narita H, Tago S, Fujita N, Harayama S (2006) Sequence analysis of three plasmids harboured in Rhodococcus erythropolis strain PR4. Environ Microbiol 8(2):334–346. https://doi.org/10.1111/j.1462-2920.2005.00899.x
Šepič E, Bricelj M, Leskovsˇek H (1997) Biodegradation studies of polyaromatic hydrocarbons in aqueous media. J Appl Microbiol 83:561–568. https://doi.org/10.1046/j.1365-2672.1997.00261.x
Shaoping K, Zhiwei D, Bingchen W, Huihui W, Jialiang L, Hongbo S (2021) Changes of sensitive microbial community in oil polluted soil in the coastal area in Shandong, China for ecorestoration. Ecotoxicol Environ Saf 207:111551. https://doi.org/10.1016/j.ecoenv.2020.111551
Sherry A, Gray ND, Ditchfield AK, Aitken CM, Jones DM, Röling WFM, Hallmann C, Larter SR, Bowler BFJ, Head IM (2013) Anaerobic biodegradation of crude oil under sulphate-reducing conditions leads to only modest enrichment of recognized sulphate-reducing taxa. Int Biodeter Biodegr 81:105–113. https://doi.org/10.1016/j.ibiod.2012.04.009
Shigenaka G (2014) Twenty-five years after the Exxon Valdez oil spill-NOAA’s scientific support, monitoring, and research. NOAA Office of Response and Restoration, Seattle, 78 pp
Shinoda Y, Sakai Y, Ué M, Hiraishi A, Kato N (2000) Isolation and characterization of a new denitrifying Spirillum. Appl Environ Microbiol 66(4):1286–1291. https://doi.org/10.1128/AEM.66.4.1286-1291.2000
Shinoda Y, Sakai Y, Uenishi H, Uchihashi Y, Hiraishi A, Yukawa H, Yurimoto H, Kato N (2004) Aerobic and anaerobic toluene degradation by a newly isolated denitrifying bacterium, Thauera sp. strain DNT-1. Appl Environ Microbiol 70(3):1385–1392. https://doi.org/10.1128/AEM.70.3.1385-1392.2004
Singh SN, Kumari B, Mishra S (2012) Microbial degradation of alkanes. Microbial Degradation of Xenobiotics, Environmental Science and Engineering, pp 439–469. https://doi.org/10.1007/978-3-642-23789-8_17
Book Google Scholar
Singleton DR, Ramirez LG, Aitken MD (2009) Characterization of a polycyclic aromatic hydrocarbon degradation gene cluster in a phenanthrene-degrading Acidovorax strain. Appl Environ Microbiol 75(9):2613–2620. https://doi.org/10.1128/AEM.01955-08
Sivaram AK, Logeshwaran P, Lockington R, Naidu R, Megharaj M (2019) Low molecular weight organic acids enhance the high molecular weight polycyclic aromatic hydrocarbons degradation by bacteria. Chemosphere 222:132–140. https://doi.org/10.1016/j.chemosphere.2019.01.110
Soleimani M, Farhoudi M, Christensen JH (2013) Chemometric assessment of enhanced bioremediation of oil contaminated soils. J Hazard Mater 254–255:372–381. https://doi.org/10.1016/j.jhazmat.2013.03.004
Song M, Yang Y, Jiang L, Hong Q, Zhang D, Shen Z, Yin H, Luo C (2017) Characterisation of the phenanthrene degradation-related genes and degrading ability of a newly isolated copper-tolerant bacterium. Environ Pollut 220(Pt B): 1059–1067. https://doi.org/10.1016/j.envpol.2016.11.037
Song XH, Xu Y, Li GM, Zhang Y, Huang TW, Hu Z (2011) Isolation, characterization of Rhodococcus sp. P14 capable of degrading high-molecular-weight polycyclic aromatic hydrocarbons and aliphatic hydrocarbons. Mar Pollut Bull 62(10):2122–2128. https://doi.org/10.1016/j.marpolbul.2011.07.013
Southam G, Whitney M, Knickerbocker C (2001) Structural characterization of the hydrocarbon degrading bacteria oil-interface: implications for bioremediation. Int Biodeter Biodegr 47:197–201. https://doi.org/10.1016/S0964-8305(01)00051-8
Stucki G, Alexander M (1987) Role of dissolution rate and solubility in biodegradation of aromatic compounds. Appl Environ Microbiol 53:292–297. https://doi.org/10.1128/aem.53.2.292-297.1987
Suganthi SH, Murshid S, Sriram S, Ramani K (2018) Enhanced biodegradation of hydrocarbons in petroleum tank bottom oil sludge and characterization of biocatalysts and biosurfactants. J Environ Manage 220:87–95. https://doi.org/10.1016/j.jenvman.2018.04.120
Sun W, Dong Y, Gao P, Fu M, Ta K, Li J (2015a) Microbial communities inhabiting oil-contaminated soils from two major oilfields in Northern China: implications for active petroleum-degrading capacity. J Microbiol 53(6):371–378. https://doi.org/10.1007/s12275-015-5023-6
Sun YM, Ning ZG, Yang F, Li XZ (2015b) Characteristics of newly isolated Geobacillus sp. ZY-10 degrading hydrocarbons in crude oil. Pol J Microbiol 64(3):253–263
Swannell RPJ, Lee K, Mcdonagh M (1996) Field evaluations of marine oil spill bioremediation. Microbiol Rev 60(2):342–365. https://doi.org/10.1128/mr.60.2.342-365.1996
Tao K, Zhang X, Chen X, Liu X, Hu X, Yuan X (2019) Response of soil bacterial community to bioaugmentation with a plant residue-immobilized bacterial consortium for crude oil removal. Chemosphere 222:831–838. https://doi.org/10.1016/j.chemosphere.2019.01.133
Tapilatu Y, Acquaviva M, Guigue C, Miralles G, Bertrand JC, Cuny P (2010) Isolation of alkane-degrading bacteria from deep-sea Mediterranean sediments. Lett Appl Microbiol 50(2):234–236. https://doi.org/10.1111/j.1472-765X.2009.02766.x
Teng Y, Luo Y, Sun M, Liu Z, Li Z, Christie P (2010) Effect of bioaugmentation by Paracoccus sp. strain HPD-2 on the soil microbial community and removal of polycyclic aromatic hydrocarbons from an aged contaminated soil. Bioresour. Technol 101(10):3437–3443. https://doi.org/10.1016/j.biortech.2009.12.088
Thangaraj K, Kapley A, Purohit HJ (2008) Characterization of diverse Acinetobacter isolates for utilization of multiple aromatic compounds. Bioresour Technol 99(7):2488–2494. https://doi.org/10.1016/j.biortech.2007.04.053
Taylor E, Reimer D (2008) Oil persistence on beaches in Prince William Sound—a review of SCAT surveys conducted from 1989 to 2002. Mar Pollut Bull 56(3):458–474. https://doi.org/10.1016/j.marpolbul.2007.11.008
Thavasi R, Jayalakshmi S, Balasubramanian T, Banat IM (2006) Biodegradation of crude oil by nitrogen fixing marine bacteria Azotobacter chroococcum. Res J Microbiol 1(5):401–408. https://doi.org/10.3923/jm.2006.401.408
Throne-Holst M, Markussen S, Winnberg A, Ellingsen TE, Kotlar HK, Zotchev SB (2006) Utilization of n-alkanes by a newly isolated strain of Acinetobacter venetianus: the role of two AlkB-type alkane hydroxylases. Appl Microbiol Biotechnol 72(2):353–360. https://doi.org/10.1007/s00253-005-0262-9
Throne-Holst M, Wentzel A, Ellingsen TE, Kotlar HK, Zotchev SB (2007) Identification of novel genes involved in long-chain n-alkane degradation by Acinetobacter sp. strain DSM 17874. Appl Environ Microbiol 73(10):3327–3332. https://doi.org/10.1128/AEM.00064-07
Turner DA, Pichtel J, Rodenas Y, McKillip J, Goodpaster JV (2015) Microbial degradation of gasoline in soil: effect of season of sampling. Forensic Sci Int 251:69–76. https://doi.org/10.1016/j.forsciint.2015.03.013
Uad I, Silva-Castro GA, Pozo C, González-López J, Calvo C (2010) Biodegradative potential and characterization of bioemulsifiers of marine bacteria isolated from samples of seawater, sediment and fuel extracted at 4000 m of depth (Prestige wreck). Int Biodeterior Biodegr 64(6):511–518. https://doi.org/10.1016/j.ibiod.2010.06.005
Varjani SJ (2017) Microbial degradation of petroleum hydrocarbons. Bioresour Technol 223:277–286. https://doi.org/10.1016/j.biortech.2016.10.037
Varjani SJ, Thaker MB, Upasani VN (2014) Optimization of growth conditions of native hydrocarbon utilizing bacterial consortium “HUBC” obtained from petroleum pollutant contaminated sites. Indian J App Res 4(10):474–476
Varjani SJ, Upasani VN (2016) Biodegradation of petroleum hydrocarbons by oleophilic strain of Pseudomonas aeruginosa NCIM 5514. Bioresour Technol 222:195–201. https://doi.org/10.1016/j.biortech.2016.10.006
Varjani SJ, Upasani VN (2016b) Carbon spectrum utilization by an indigenous strain of Pseudomonas aeruginosa NCIM 5514: production, characterization and surface active properties of biosurfactant. Bioresour Technol 221:510–516. https://doi.org/10.1016/j.biortech.2016.09.080
Varjani SJ, Upasani VN (2016a) Core flood study for enhanced oil recovery through ex-situ bioaugmentation with thermo- and halo-tolerant rhamnolipid produced by Pseudomonas aeruginosa NCIM 5514. Bioresour. Technol. 220:175–182. https://doi.org/10.1016/j.biortech.2016.08.060
Varjani SJ, Upasani VN (2017) A new look on factors affecting microbial degradation of petroleum hydrocarbon pollutants. Int Biodeter Biodegr 120:71–83. https://doi.org/10.1016/j.ibiod.2017.02.006
Venosa AD, Haines JR, Allen DM (1992) Efficacy of commercial inocula in enhancing biodegradation of weathered crude oil contaminating a Prince William Sound beach. J Ind Microbiol 10:1–11. https://doi.org/10.1007/BF01583628
Venosa AD, Suidan MT, Wrenn BA, Strohmeier KL, Haines JR, Eberhart BL, King D, Holder E (1996) Bioremediation of an exprimental oil spill on the shoreline of Delaware Bay. Environ Sci Technol 30(5):1764–1775. https://doi.org/10.1021/es950754r
Venosa AD, Zhu XQ (2003) Biodegradation of crude oil contaminating marine shorelines and freshwater wetlands. Spill Sci Technol B 8(2):163–178. https://doi.org/10.1016/S1353-2561(03)00019-7
Vila J, Maria Nieto J, Mertens J, Springael D, Grifoll M (2010) Microbial community structure of a heavy fuel oil-degrading marine consortium: linking microbial dynamics with polycyclic aromatic hydrocarbon utilization. FEMS Microbiol Ecol 73(2):349–362. https://doi.org/10.1111/j.1574-6941.2010.00902.x
Walworth J, Pond A, Snape I, Rayner J, Ferguson S, Harvey P (2007) Nitrogen requirements for maximizing petroleum bioremediation in a sub-Antarctic soil. Cold Reg Sci Technol 48(2):84–91. https://doi.org/10.1016/j.coldregions.2006.07.001
Wang H, Gilbert JA, Zhu Y, Yang X (2018a) Salinity is a key factor driving the nitrogen cycling in the mangrove sediment. Sci Total Environ 631–632:1342–1349. https://doi.org/10.1016/j.scitotenv.2018.03.102
Wang L, Wang W, Lai Q, Shao Z (2010) Gene diversity of CYP153A and AlkB alkane hydroxylases in oil-degrading bacteria isolated from the Atlantic Ocean. Environ Microbiol 12(5):1230–1242. https://doi.org/10.1111/j.1462-2920.2010.02165.x
Wang W, Wang L, Shao Z (2018b) Polycyclic aromatic hydrocarbon (PAH) degradation pathways of the obligate marine PAH degrader Cycloclasticus sp. strain P1. Appl Environ Microbiol 84(21). https://doi.org/10.1128/AEM.01261-18
Wang WP, Shao ZZ (2012) Diversity of flavin-binding monooxygenase genes (almA) in marine bacteria capable of degradation long-chain alkanes. FEMS Microbiol Ecol 80(3):523–533. https://doi.org/10.1111/j.1574-6941.2012.01322.x
Wang X, Liu Y, Song C, Yuan X, Zhang Q, Miao Y (2020a) Application analysis of immobilized bioremediation preparation in oil spill contaminated shore. IOP Conference Series: Earth and Environmental Science 558:042029. https://doi.org/10.1088/1755-1315/558/4/042029
Wang X, Sun L, Wang H, Wu H, Chen S, Zheng X (2018c) Surfactant-enhanced bioremediation of DDTs and PAHs in contaminated farmland soil. Environ Technol 39(13):1733–1744. https://doi.org/10.1080/09593330.2017.1337235
Wang Z, An CJ, Lee K, Owens E, Chen Z, Boufadel M, Taylor E, Feng Q (2020b) Factors influencing the fate of oil spilled on shorelines: a review. Environ Chem Lett 19(2):1611–1628. https://doi.org/10.1007/s10311-020-01097-4
Wang ZD (2007) Oil spill environmental forensics: fingerprinting and source identification.
Weelink SAB, van Eekert MHA, Stams AJM (2010) Degradation of BTEX by anaerobic bacteria: physiology and application. Rev Environ Sci Bio 9(4):359–385. https://doi.org/10.1007/s11157-010-9219-2
Wentzel A, Ellingsen TE, Kotlar HK, Zotchev SB, Throne-Holst M (2007) Bacterial metabolism of long-chain n-alkanes. Appl Microbiol Biotechnol 76(6):1209–1221. https://doi.org/10.1007/s00253-007-1119-1
Widdel F, Rabus R (2001a) Anaerobic biodegradation of saturated and aromatic hydrocarbons. Curr Opin Biotech:12. https://doi.org/10.1016/S0958-1669(00)00209-3
Widdel F, Rabus R (2001b) Anaerobic biodegradation of saturated and aromatic hydrocarbons. Curr Opin Biotechnol 12(3):259–276. https://doi.org/10.1016/S0958-1669(00)00209-3
Wu RR, Dang Z, Yi XY, Yang C, Lu GN, Guo CL, Liu CQ (2011) The effects of nutrient amendment on biodegradation and cytochrome P450 activity of an n-alkane degrading strain of Burkholderia sp. GS3C. J Hazard Mater 186(2-3):978–983. https://doi.org/10.1016/j.jhazmat.2010.11.095
Xia MQ, Liu Y, Taylor AA, Fu DF, Khan AR, Terry N (2017) Crude oil depletion by bacterial strains isolated from a petroleum hydrocarbon impacted solid waste management site in California. Int Biodeterior Biodegr 123:70–77. https://doi.org/10.1016/j.ibiod.2017.06.003
Xia W, Du Z, Cui Q, Dong H, Wang F, He P, Tang Y (2014) Biosurfactant produced by novel Pseudomonas sp. WJ6 with biodegradation of n-alkanes and polycyclic aromatic hydrocarbons. J Hazard Mater 276:489–498. https://doi.org/10.1016/j.jhazmat.2014.05.062
Xu X, Chen X, Su P, Fang F, Hu B (2016) Biodegradation potential of polycyclic aromatic hydrocarbons by bacteria strains enriched from Yangtze River sediments. Environ Technol 37(5):513–520. https://doi.org/10.1080/09593330.2015.1074289
Xu X, Liu W, Tian S, Wang W, Qi Q, Jiang P, Gao X, Li F, Li H, Yu H (2018) Petroleum hydrocarbon-degrading bacteria for the remediation of oil pollution under aerobic conditions: a perspective analysis. Front Microbiol 9:2885. https://doi.org/10.3389/fmicb.2018.02885
Xue J, Yu Y, Bai Y, Wang L, Wu Y (2015) Marine oil-degrading microorganisms and biodegradation process of petroleum hydrocarbon in marine environments: a review. Curr Microbiol 71(2):220–228. https://doi.org/10.1007/s00284-015-0825-7
Yakimov MM, Giuliano L, Denaro R, Crisafi E, Chernikova TN, Abraham WR, Luensdorf H, Timmis KN, Golyshin PN (2004) Thalassolituus oleivorans gen. nov., sp. nov., a novel marine bacterium that obligately utilizes hydrocarbons. Int J Syst Evol Microbiol 54(Pt 1):141–148. https://doi.org/10.1099/ijs.0.02424-0
Yakimov MM, Giuliano L, Gentile G, Crisafi E, Chernikova TN, Abraham WR, Lunsdorf H, Timmis KN, Golyshin PN (2003) Oleispira antarctica gen. nov., sp. nov., a novel hydrocarbonoclastic marine bacterium isolated from Antarctic coastal sea water. Int J Syst Evol Microbiol 53(Pt 3):779–785. https://doi.org/10.1099/ijs.0.02366-0
Yuste L, Corbella ME, Turiégano MJ, Karlson U, Puyet A, Rojo F (2000) Characterization of bacterial strains able to grow on high molecular mass residues from crude oil processing. FEMS Microbiol Ecol 32:69–75. https://doi.org/10.1111/j.1574-6941.2000.tb00700.x
Yuewen D, Adzigbli L (2018) Assessing the impact of oil spills on marine organisms. J Oceanogr Mar Res 6:472–479
Zahed MA, Salehi S, Madadi R, Hejabi F (2021) Biochar as a sustainable product for remediation of petroleum contaminated soil. Curr Res Green Sust Chem 4:100055. https://doi.org/10.1016/j.crgsc.2021.100055
Zeng J, Zhu Q, Wu Y, Chen H, Lin X (2017) Characterization of a polycyclic aromatic ring-hydroxylation dioxygenase from Mycobacterium sp. NJS-P. Chemosphere 185:67–74. https://doi.org/10.1016/j.chemosphere.2017.07.001
Zengler K, Heider J, Rosselló-Mora R, Widdel F (1999) Phototrophic utilization of toluene under anoxic conditions by a new strain of Blastochloris sulfoviridis. Arch Microbiol 172:204–212. https://doi.org/10.1007/s002030050761
Zhang B, Matchinski EJ, Chen B, Ye X, Jing L, Lee K (2019a) Marine oil spills—oil pollution, sources and effects. 391–406. https://doi.org/10.1016/B978-0-12-805052-1.00024-3
Zhang J, Lin XG, Liu WW, Wang YM, Zeng J, Chen H (2012) Effect of organic wastes on the plant-microbe remediation for removal of aged PAHs in soils. J Environ Sci (China) 24(8):1476–1482. https://doi.org/10.1016/S1001-0742(11)60951-0
Zhang S, Hu Z, Wang H (2019b) Metagenomic analysis exhibited the co-metabolism of polycyclic aromatic hydrocarbons by bacterial community from estuarine sediment. Environ Int 129:308–319 https://doi.org/10.1016/j.envint.2019.05.028
Zhao F, Zhou JD, Ma F, Shi RJ, Han SQ, Zhang J, Zhang Y (2016) Simultaneous inhibition of sulfate-reducing bacteria, removal of H2S and production of rhamnolipid by recombinant Pseudomonas stutzeri Rhl: applications for microbial enhanced oil recovery. Bioresour Technol 207:24–30. https://doi.org/10.1016/j.biortech.2016.01.126
Zheng C, He J, Wang Y, Wang M, Huang Z (2011) Hydrocarbon degradation and bioemulsifier production by thermophilic Geobacillus pallidus strains. Bioresour Technol 102(19):9155–9161. https://doi.org/10.1016/j.biortech.2011.06.074
Zhong H, Liu G, Jiang Y, Yang J, Liu Y, Yang X, Liu Z, Zeng G (2017) Transport of bacteria in porous media and its enhancement by surfactants for bioaugmentation: a review. Biotechnol Adv 35(4):490–504. https://doi.org/10.1016/j.biotechadv.2017.03.009
Zhong H, Liu Y, Liu ZF, Jiang YB, Tan F, Zeng GM, Yuan XZ, Yan M, Niu QY, Liang YS (2014) Degradation of pseudo-solubilized and mass hexadecane by a Pseudomonas aeruginosa with treatment of rhamnolipid biosurfactant. Int Biodeter Biodegra 94:152–159. https://doi.org/10.1016/j.ibiod.2014.07.012
Zhou L, Li H, Zhang Y, Han S, Xu H (2016) Sphingomonas from petroleum-contaminated soils in Shenfu, China and their PAHs degradation abilities. Braz J Microbiol 47(2):271–278. https://doi.org/10.1016/j.bjm.2016.01.001
Zhu X, Jin L, Sun K, Li S, Ling W, Li X (2016) Potential of endophytic bacterium Paenibacillus sp. PHE-3 isolated from Plantago asiatica L. for reduction of PAH contamination in Plant Tissues. Int J Environ Res Public Health 13(7). https://doi.org/10.3390/ijerph13070633
Zhuang WQ, Tay JH, Maszenan A, Tay S (2002) Bacillus naphthovorans sp. nov. from oil-contaminated tropical marine sediments and its role in naphthalene biodegradation. Appl. Microbiol. Biotechnol 58:547–554
Download references
The work is financially supported by the National Key Research and Development Program of China (No. 2022YFC3203001) and the Sprout Project of Beijing Academy of Science and Technology (No. 2022A-0006).
Author information
Xiaoli Dai, Jing Lv, and Pengcheng Fu contributed equally to this work.
Authors and Affiliations
Beijing Key Laboratory of Remediation of Industrial Pollution Sites, Institute of Resources and Environment, Beijing Academy of Science and Technology, Beijing, 10089, China
China University of Petroleum-Beijing, Beijing, 102249, China
Jing Lv & Shaohui Guo
State Key Laboratory of Marine Resource Utilization in South China Sea, Hainan University, Hainan, 570228, China
Pengcheng Fu
You can also search for this author in PubMed Google Scholar
Contributions
XLD: writing—original draft, conceptualization, and resources; JL: writing—review and editing and resources; SHG: writing—review and editing; PCF: writing—review and editing, conceptualization, methodology, and supervision.
Corresponding author
Correspondence to Xiaoli Dai .
Ethics declarations
Ethics approval and consent to participate, consent for publication, competing interests.
The authors declare no competing interests.
Additional information
Responsible Editor: Robert Duran
Publisher’s note
Springer Nature remains neutral with regard to jurisdictional claims in published maps and institutional affiliations.
Rights and permissions
Springer Nature or its licensor (e.g. a society or other partner) holds exclusive rights to this article under a publishing agreement with the author(s) or other rightsholder(s); author self-archiving of the accepted manuscript version of this article is solely governed by the terms of such publishing agreement and applicable law.
Reprints and permissions
About this article
Dai, ., Lv, J., Fu, P. et al. Microbial remediation of oil-contaminated shorelines: a review. Environ Sci Pollut Res 30 , 93491–93518 (2023). https://doi.org/10.1007/s11356-023-29151-y
Download citation
Received : 27 March 2023
Accepted : 31 July 2023
Published : 12 August 2023
Issue Date : September 2023
DOI : https://doi.org/10.1007/s11356-023-29151-y
Share this article
Anyone you share the following link with will be able to read this content:
Sorry, a shareable link is not currently available for this article.
Provided by the Springer Nature SharedIt content-sharing initiative
- Oil pollution
- Microbial remediation
- Environmental adaptability
- Sustainable microbial remediation
- Find a journal
- Publish with us
- Track your research
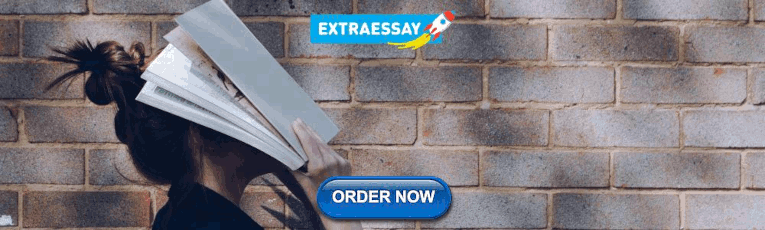
IMAGES
VIDEO
COMMENTS
Nano-enhanced bioremediation is a new field of study, and more research is undeniably needed. The interdisciplinary combination offers new opportunities and novel functionalities such as. 1. Sustainable and environmentally friendly materials that can be used as alternatives to traditional post oil spill response.
of GEM to oil spill bioremediation has the potential to. greatly accelerate the biodegradation of spilled. petroleum if it can be safely applied. A single bioremediation appr oach may be incapable ...
The devastating environmental impacts of the Exxon Valdez spill in 1989 and its media notoriety made it a frequent comparison to the BP Deepwater Horizon spill in the popular press in 2010, even though the nature of the two spills and the environments impacted were vastly different. Fortunately, unlike higher organisms that are adversely impacted by oil spills, microorganisms are able to ...
Metrics. A desert soil sample was saturated with crude oil (17.3%, w/w) and aliquots were diluted to different extents with either pristine desert or garden soils. Heaps of all samples were ...
All the papers selected were published in English language from 2000 to 2021. 29 papers are studies on biological methods, 10 papers investigated the effectiveness of combining different soil remediation methods in oil spill removal, while 7 papers are on chemical remediation methods, and the remaining 5 papers studied the effectiveness of ...
Although substantial research on oil bioremediation has been undertaken over the last decade, the majority of already available studies focused on assessing the use of bioremediation in the oil spills around the globe. Only a few experiments have been done and tested, and the results are quite extraordinary.
Bioremediation technologies, on the other hand, have been considered promising ecologic alternatives to tackle oil spills, avoiding the negative implications associated with physical-chemical techniques, like the introduction of chemical dispersants or burning the spilled oil [4,5,6]. Bioremediation can be divided into two strategies ...
This natural process has shown to be a successful way of oil spill cleanup in following years. It has even been proposed that bioremediation of oil spills is the only large-scale cleanup procedure that can be handled effectively (Snape et al., 2001). Bioremediation offers several benefits over traditional ways of cleaning up marine oil spills.
This review outlines the advancements made for bioremedia-tion and nanoscience for oil spill remediation. Nano-enhanced bioremediation is a new eld of study, and more research is. fi. undeniably needed. The interdisciplinary combination o ers. ff. new opportunities and novel functionalities such as.
Abstract. Oil spills are probable accidents occurring mostly during transportation and processing of oil that can contaminate marine, soil, sediments, and other environments. Oil spill is a special challenge to be remediated due to its several environmental, economic, and social threats. Several physical (mechanical), chemical, and biological ...
As the demand for liquid petroleum increases, the need for reliable and efficient oil spill clean-up techniques is inevitable. Bioremediation is considered one of the most sustainable clean-up techniques but the potential has not been fully exploited in the field because it is too slow to meet the immediate demands of the environment. This study reviews the challenges to managing oil spills in ...
Bioremediation of oil spills: A review of challenges for research advancement. As the demand for liquid petroleum increases, the need for reliable and efficient oil spill clean-up techniques is inevitable. Bioremediation is considered one of the most sustainable clean-up techniques but the potential has not been fully exploited in the field ...
Abstract. Spills of crude and fuel oils from tankers, freighters, pipelines, wells, and storage facilities into the marine environment capture the public's attention and demand prompt and environmentally sensitive response technologies. Sometimes it is possible to contain the oil with booms and collect it with skimmers or burn it, but in many ...
Bioremediation studies mentioned in this paper can be used for treatment such as emulsified residual spilled oil in seawater with floating oil spill containment booms as an enclosed basin such as a bioreactor, for petroleum hydrocarbons as a pollutant that will help environmental researchers solve these problems and completely clean-up oil ...
The technologies to remove contaminants from a polluted site can be grouped into two main categories and applied individually or in synergy: physicochemical technologies, and biological technologies (bioremediation) (Alvarez and Illman, 2006).Physicochemical technologies can include physical removal (i.e. excavation of soil and sediment or groundwater pumping), washing by co-solvents or ...
Agricultural wastes, such as wheat bran and swine wastewater, were used for bioremediation of oil-contaminated soil. Two optimised strains that could degrade oil efficiently were selected. The ...
Abstract — Bioremediation of Marine Oil Spills — In the long run, biodegradation is the eventual fate. of oil spilled at sea that cannot be collected or burnt. Stimulating this biodegradation ...
The future research on the oil spill bioremediation must be focused on these new aspects of the process and finally pave the way for application of bioremediation in real field to obtain promising ...
As the demand for liquid petroleum increases, the need for reliable and efficient oil spill clean-up techniques is inevitable. Bioremediation is considered one of the most sustainable clean-up techniques but the potential has not been fully exploited in the field because it is too slow to meet the immediate demands of the environment. This study reviews the challenges to managing oil spills in ...
A new joint colloquium report from the American Academy of Microbiology (AAM), the American Geophysical Union (AGU), and the Gulf of Mexico Research Initiative (GoMRI) titled "Microbial Genomics of the Global Ocean System reports on science findings in the 10 years since the Deepwater Horizon (DWH) oil spill, which dumped 4.9 million barrels of oil and 250,000 metric tons of natural gas into ...
Research institutes, oil firms, government/environmental agencies and NGOs may help provide research funds for field studies as this would produce more reliable scientific reports on the potentials of the proposed bioremediation strategies and consequently improve oil spill management techniques for terrestrial and aquatic environments.
Bioremediation is a cost-effective and practical solution for removing environmental contaminants . Plant growth promotion, insect control, soil conservation, nutrient recycling, and pollutant reduction are all key functions of soil microorganisms . Bioremediation has come a long way in terms of efficiency, cost, and social acceptability ...
Frequent marine oil spills have led to increasingly serious oil pollution along shorelines. Microbial remediation has become a research hotspot of intertidal oil pollution remediation because of its high efficiency, low cost, environmental friendliness, and simple operation. Many microorganisms are able to convert oil pollutants into non-toxic substances through their growth and metabolism ...
Global warming-induced sea ice loss in the Canadian Northwest Passage (NWP) will result in more shipping traffic, increasing the risk of oil spills. Microorganisms inhabiting NWP beach sediments may degrade hydrocarbons, offering a potential bioremediation strategy.
Novel production of biodispersant by Serratia marcescens UCP 1549 in solid-state fermentation and application for oil spill bioremediation. ... Showing 1 through 3 of 0 Related Papers. 59 References; ... Semantic Scholar is a free, AI-powered research tool for scientific literature, based at the Allen Institute for AI. Learn More. About About ...